Vol 8 No 3 2023 – 34
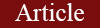
Physicochemical, Hydration and Steady Shear Rheological Properties of Flours Derived From Different Crop Residues From the Honduran Agro–Food Industry
1 Department of Agriculture and Forestry Engineering, Food Technology, College of Agricultural and Forestry Engineering, University of Valladolid, Palencia, Spain.
2 Department of Agroindustrial Engineering, Pacific Littoral Regional University Center, National Autonomous University of Honduras (UNAH), Choluteca, Honduras.
3 Scientific Research Management Unit, Agroindustrial Engineering, National Autonomous University of Honduras Technological Danlí, Danlí, Honduras.
* Correspondence: caleb.calix@unah.edu.hn;
Available from: http://dx.doi.org/10.21931/RB/2023.08.03.34
ABSTRACT
This study aimed to determine the physicochemical, hydration, and rheological characteristics of flours derived from different crop residues (taro, cassava, maize, and banana) affected by climate change in Honduras and to investigate their potential application in food processes. The physicochemical (moisture content, ash, protein, crude fat, starch, amylose content, and color), hydration (water absorption capacity (WAC), water absorption index (WAI), water solubility index (WSI), swelling power (SP), true and bulk density), pasting (pasting temperature, peak, trough, breakdown, setback, and final viscosity), and rheological properties of the flours were evaluated. Taro flour had the highest values of ash content, WAI and SP and the lowest for crude fat, amylose content, bulk density and peak, breakdown, setback viscosity and consistency index. Cassava flour had the highest breakdown viscosity and flow behavior index values and the lowest for protein content, hysteresis loop area, yield stress, and consistency index. Maize flour had the highest crude fat, protein, and amylose content, bulk density, WAC, setback viscosity, hysteresis loop area, and yield stress, and the lowest values were for ash, protein content, WSI, pasting temperature, breakdown viscosity, and flow behavior index. Banana flour had the highest starch, carbohydrate, WSI, pasting temperature, peak viscosity and consistency index, and the lowest value for protein content, WAI and SP. The study’s results have determined the compositional value and functionality of the flours obtained from the agro-residues studied for their potential use in different food products.
Keywords: Crop residues flour; Food security; Change climatic; physicochemical characterization; steady shear
INTRODUCTION
Food and nutrition security is a global challenge that aims to ensure that all people, at all times, have economic and physical access to sufficient, safe, and nutritious food1. The importance of food loss and food waste has increased over the last few decades and was included within the United Nations 2015 Sustainable Development Goals (SDGs), with particular reference to zero hunger (SDG 2) and responsible consumption and production (SDG 12). By 2030, humans must «halve per capita global food waste at the retail and consumer levels and reduce food losses along production and supply chains, including post-harvest losses»2,3. Roughly one-third of the edible parts of global food produced for human consumption is estimated to be lost or wasted, and these losses have been valued at 1 trillion USD 4. Honduras has a population of almost 10 million inhabitants, facing a complex social and economic reality, and is one of the poorest and most unequal countries in America. It is also located in Central America’s ‘Dry Corridor’ area, which is particularly susceptible to irregular and long-lasting droughts5. The past two years (2020–2022) have delivered multiple overlapping challenges to food security, including climate change, conflicts, and the COVID-19 pandemic.
Furthermore, in addition to the issues above, the country was affected by the hurricanes Eta and Iota in November 2020. It is projected to be disproportionately impacted by volatility in food, agricultural input, and energy prices related to tensions between Russia and Ukraine6. The Honduran population is more than 50% rural and thus highly dependent on the crops and natural resources that extreme weather events can decimate. As such, the Notre Dame Global Adaptation Initiative ranks Honduras as the 69th most vulnerable country to climate change and one of the world’s least ‘ready’ nations regarding adaptation capacity7. Documented climatic changes in Honduras since 1960 include an average annual temperature increase of 0.6 degrees Celsius, increased heavy rainfall events of 1.2% per decade, more significant inconsistency in yearly rainfall, and a longer and hotter dry season (canícula)8. Climate change may affect the country’s agro-industry, generating crop losses (40-100%), reduction of water flows, water shortages, the appearance of new and stronger pests, and economic losses, thus generating a situation of food insecurity. Compounding the challenge, Honduras suffers from the double burden of malnutrition and obesity5. Crops such as taro (Colocasia esculenta), cassava (Manihot esculenta), maize (Zea mays) and banana (Musa paradisiaca) are mainly affected by this situation. Naturally, the waste from these crops is left abandoned in the fields, causing economic losses and environmental contamination. This organic residue can be an important source of nutrients. It has been suggested that they could be valued and used as ingredients or raw materials in some production processes and to develop new products9. One alternative would be adding value to agro-waste to produce flour for use in food applications, thus reducing food insecurity in the country. This study aimed to assess the physicochemical, hydration, pasting and steady shear rheological characteristics of crop residues flour from various sources affected by climatic change in Honduras, providing an approach to their possible applicability in new food products.
MATERIALS AND METHODS
Materials
The agro-waste flour samples evaluated in this study were obtained from crops ruined and affected by climate change and did not meet the quality standards for commercialization. Various producers of the SAN R11 Bureau in El Paraíso, Honduras, provided the samples. The selected native varieties for analysis included taro (Colocasia esculenta), cassava (Manihot esculenta), green banana (Musa paradisiaca), and maize (Zea mays).
Flours preparation
The collected taro, cassava and banana were sorted and cleaned with potable water to remove dust and soil from the surface and then peeled by hand. The peeled taro, cassava and banana were grated into slices with an approximate thickness between 0.1 – 0.3 cm. Subsequently, samples were dried at 60 ᵒC for 7 h in a dehydrator (Agropec, Honduras). The dried samples were ground using a lab-scale grinder and sieved using a 600 µm mesh size to get a uniform particle size. For maize, the protocol employed to obtain the flour was used for taro, cassava and banana. However, the kernels were pre-crushed before being dried using a manual hammer mill (Corona, Colombia). The flours were stored in airtight plastic packets at a cooling temperature (4 ᵒC) for further assays.
Proximal analysis of flour
The moisture and ash content were determined using method 44–19 and 08–12 of AACC10, respectively. Total nitrogen (N) in samples was evaluated by a Leco CNS 928 microanalyzer (Leco Corporation, St. Joseph, MI. USA). Protein content was calculated using the nitrogen concentration (N) with the conversion factor 6.25 (N × 6.25). Crude fat was determined by a gravimetric method using a Soxhlet apparatus with hexane as the extraction solvent, according to the official AOAC method 923.05–192311. Starch and amylose content were determined using an amylose/amylopectin assay kit (Megazyme, Wicklow, Ireland). For the yield, the samples were weighed at the beginning and end of the preparation process to obtain the relation between the amount of raw material processed and the flour sample obtained. All determinations were performed in duplicate.
Least gelation concentration
The least gelation concentration (LGC) was determined by the method of Singh et al. (2017)12 with slight modifications. The flour dispersions of 0.5, 1, 2, 4, 6, 8, 10, 12 and 14% (w/v) were prepared in 5 mL distilled water. The suspensions were vortexes and heated in a water bath at 90 ᵒC for 1 hour. The test tubes were cooled for 2 hours at 4 ± 2 ᵒC. The LGC was determined as the concentration when samples from inverted test tubes did not slip. A gel was assumed to be formed when there was no slippage after the inversion of the tubes. A soft gel was deemed to have been included when the gel flowed somewhat on inversion. If the suspension slid down, depending on the rate of descent, it was considered either a liquid or viscous.
True and Bulk densities
True density (TD) and bulk density (BD) were determined according to the methods described by Abebe, Collar and Ronda. (2015)13. TD was determined by liquid displacement method with toluene using 50 mL pycnometers. BD was evaluated by pouring the flour samples gently into previously tared 10 mL graduated cylinders. After vibrating the sample, the final volume reading was taken until a constant value. Assays were performed in duplicate.
Color of flours
A PCE-CSM5 (PCE Instruments, Meschede, Germany) and CQCS software were used for flour color measurements. Results were obtained in the CIE L, a*, b* (dimensionless) coordinates using the D65 standard illuminant and the 10◦ standard observer. Five measurements were made for each flour.
Hydration properties
Water absorption capacity, water absorption index, water solubility index and swelling power of flours were determined according to the methods described by Calix-Rivera et al. (2023)14. All results are referred to as flour dry matter. Samples were evaluated at least in triplicate.
Pasting properties by rapid visco analyzer (RVA)
Pasting properties were evaluated using a Rapid Visco Analyzer model RVA 4500 (PerkinElmer, Australia). 3 g of each flour at 14% moisture basis and 25 mL of distilled water were added into aluminum canisters and performed according to the international method AACC 76-21.0215. The initial temperature of assays was set at 50 ᵒC for 1 min, then increased to 95 ᵒC at a rate of 12.2 ᵒC/min and held for 2.5 min, subsequently reduced to 50 ᵒC at a rate of 11.8 ᵒC/min and finally held at 50 ᵒC for 2 min. Tests were performed at 160 rpm. The recorded parameters included the pasting temperature (PT), peak viscosity (PV), trough viscosity (TV), final viscosity (FV), breakdown viscosity (BV = PV – TV) and setback viscosity (SV = FV – TV). The parameters of RVA were computed by analyzing the pasting curve with the aid of ThermoCline for Windows TCW3 software (PerkinElmer, Australia). The analysis was performed in duplicate.
Steady shear analysis
The steady shear tests of flours were obtained using a rheometer Kinexus Pro+ rheometer (Malvern Instruments Ltd, UK). Flour pastes (8% w/w) were prepared following the procedure in the previous section (pasting properties). At the end of the pasting test, the gels were removed from the canister and placed between serrated parallel plate geometry (40 mm diameter) with a 1 mm working gap. The sample excess was removed, and the edge was coated with Vaseline (Panreac, Barcelona, Spain) to prevent water evaporation during the measurement. The flour pastes were conditioned at 25 ᵒC for 5 min before shearing from 0.1 to 100 s−1 (upward sweep) and then from 100 to 0.1 s−1 (downward sweep). The experimental data were fitted to the Herschel-Bulkley model (Eq. 1):

Where σ is the shear stress (Pa), σo represents the yield stress (Pa), γ is the shear rate (s−1), k is the consistency coefficient (Pa·sn), and n is the flow behavior index (dimensionless). The apparent viscosity was determined using the following equation (Eq. 2):

ηapp is the apparent viscosity, σ is the shear stress (Pa), and γ is the shear rate (s−1). The samples’ corresponding thixotropic indexes were determined. Assays were performed at least by duplicate.
Statistical analysis
The data obtained were analyzed statistically using Statgraphics Centurion XIX software (Statgraphics Technologies, Inc. Virginia, U.S.A.) to determine statistical significance. ANOVA was performed, and means were compared by Fisher’s least significant difference (LSD) test. p-value < 0.05 was considered significant.
RESULTS & DISCUSSION
Proximal analysis
Table 1. Proximal composition of flour analyzed
The yields and proximal composition of flours are presented in Table 1. The results seem to show variations in the yields of the flour process, 64.05% maize > 25.01% cassava > 21.16% taro > 15.02% banana, in decreasing order, which could be attributed to the processing method and the specific characteristics of the raw material, in agreement with Acevedo et al. (2020)16, who obtained differences in starch extraction yields from Argentinian legumes and reported that these differences could be associated with the species and cultivars of the seed and the estimation methods. Higher yields of agro-residue flours are a prerequisite to higher profitability of potential finished food products. All flours showed moisture contents <14%, which is recommended for good preservation of the flours17. The moisture content of the samples ranged from 7.58 ± 0.01 to 11.67 ± 0.01% for taro and maize, respectively. According to the obtained results, there were significant differences (p < 0.05) between the analyzed samples. The moisture content of taro and maize reported by Rodriguez-Miranda et al. (2011)18, whose values were 6.22 ± 0.06% and 10.61 ± 0.11%, respectively, were lower than those obtained in this study, possibly because of the processing of the samples studied. The moisture results for cassava and banana flour were similar to those reported by Tambo Tene et al. (2019)17 and Abioye et al. (2011)19, respectively (bitter cassava flour: 12.55 ± 0.40% and banana flour: 9.65 ± 0.03%). Maize flour showed the highest protein (7.35 ± 0.12%) and fat (4.4 ± 0.9%) content, followed by taro flour, cassava and plantain flour (these with no significant differences). These results, particularly the protein content, could be related to the type of sample studied. Likewise, Tambo Tene et al. (2019)17 attributed differences in the results to the variety, locality where it was harvested, cultivation practices, and chemical composition of the soil. Similarly, Rodriguez-Miranda et al. (2011)18 found higher fat and protein values in maize flour than in taro flour, noting that cereals generally have a higher content of these components than tubers. This is important because a possible mixture of these flours could increase the nutritional value of the resulting products (Rodriguez-Miranda et al. 2011)18. Ash content ranged from 0.53 ± 0.01% (maize) to 3.39 ± 0.12% (taro), with significant differences (p < 0.05) between flours. Taro flour exhibited a considerably elevated ash content compared to the other types of flour. Rodríguez-Miranda et al. (2011)18 reported a comparable ash content in taro flour used to produce extruded snacks. They attributed this finding to the presence of calcium oxalate crystals, abundant in the Araceae family to which taro belongs and are known to contribute to ash formation. No significant differences (p > 0.05) were observed between the samples for total starch. Our results showed higher total starch values than those reported by other studies: taro (41.27 – 64.44%)20; cassava (70.48 – 82.42%)21; maize ( 60.1 – 75.2%)22; banana (60.12 – 97.26%)23; These variations could be attributed to the determination method. Amylose content (AC) determines many functional properties of starch, including gelatinization, pasting, gelling, and retrogradation/syneresis24. Maize flour exhibited the highest AC (23 ± 1%), followed by cassava (18.4 ± 0.5%) and banana (17.7 ± 0.3%) flours, and finally taro flour with 13 ± 1%. Hoover (2011)25 reported lower AC for taro flour (21.4%) and similar AC for cassava (18.6 – 23.6%); however, the AC written by Oyeyinka et al. (2019)26 for cassava flour (22 – 27%) was higher than that obtained in this study. AC may be associated with genetic differences inherent to the plant species, botanical origin, plant physiological state, crop maturation and environmental growth conditions 24,27. Starch or flour with a relatively large concentration of amylose is chosen to produce clear noodles and «rice» sticks, which require a solid starch-gelling ability of the starch24.
Least gelation concentration
The leastt gelation concentrations results of samples are summarized in Table 2. The ability of flours to form gels on heating is an essential functional property in food processing, and it occurs when starch and proteins form a 3D network that is opposed to flowing under pressure12. Starch’s ability to create gels and provide a structural matrix for holding flavors, sugars, water and food ingredients is essential in food formulation and developing new products28. Taro and banana formed gel at relatively low concentrations (2% w/v). Low values of LGC for taro and banana indicate the better gelation ability of the protein ingredient and the swelling capacity of the flours29, which may be an asset for the formulation of curd or as an additive to other gel-forming materials in food products29.
Table 2. Least gelation concentration of flour
Maize and cassava formed gel at higher concentrations (4% w/v); in the case of maize, the presence of a high amount of lipids, as can be seen in Table 1, could have a high impact on the swelling power of starch and hence its lower gelling ability. High values of LGC could have an advantage in preparing weaning foods 12. Different values of LGC in samples could be due to the nature of the starch 30. Furthermore, the type and content of proteins, carbohydrates and lipids, and the interactions among them 31 suggest that the interaction between these components may also have a significant role in functional properties. Overall, the LGC of the maize and cassava flours was higher than that of taro and banana flours; hence, less taro and banana flours would be required to form pastes during food processing compared to maize and cassava flours. It may be an economic advantage to use them.
Bulk and true density
The bulk and true densities of the samples are included in Table 3. TD showed significant differences and varied in the following order: cassava flour (1.425 g/ml) < maize flour (1.443 g/ml) < banana flour (1.459 g/ml) < taro flour (1.485 g/ml).
Table 3. Bulk and proper density, color, hydration properties and pasting properties of flours studied
This could be related to other physical properties, such as particle size and their different compositions13. The results of BD ranged in value from 0.765 g/ml to 0.819 g/ml. The taro flour had a BD of 0.765 g/ml, comparable with values reported by Mbofung et al. (2006)20, which ranged from 0.57 to 0.71 g/ml of six taro varieties and observed significant correlations between the constituents (available carbohydrates, starch, and amylose content), functional properties, foaming capacity, bulk density, and starch. The BD of cassava flour (0.776 g/ml) was similar to values reported by Hasmadi et al. (2020)32, who reported density values from 0.57 g/ml to 0.79 g/ml for 2 cassava flours grown in two different geographical locations and reported that bulk density is generally affected by the particle size and actual thickness of the flour and is one of the essential parameters for determining the type of packaging material, handling and application in the food industry. The higher BD value of 0.819 g/ml observed in maize flour coincides with the findings by Bolade et al. (2009)33, whose reported BD values ranging from 0.80 to 0.93 g/ml for 300 – 425 µm flour fractions and 0.84 g/ml for < 425 µm whole maize flour fractions. The BD of banana flour in this study was similar to those reported by Pragati et al. (2014)34, with values ranging from 0.67 to 0.72 g/ml. Low densities like taro and cassava flours could be recommended to prepare infant flours17. Marcel et al. (2021)35 characterized banana/plantain composite flours with different substitution levels of maize flour for making doughnuts and reported density values between 0.75 g/ml – 0.74 g/ml with 5% and 10% maize flour in the mix, respectively. Marcel et al. (2021)35 noted that banana and plantain composite flours produced in their study could find use as a food thickener in the food industries to give a body and mouth feel to food products. They revealed that using banana-maize or plantain-maize composite flour in preparing pastries, especially doughnuts depends on their interaction with water and oil during rehydration or frying.
Color characteristics
The results of the color analysis are shown in Table 3. Color is an essential organoleptic quality attribute that typically relates to food products’ acceptability, marketability and wholesomeness 21,36,37. The color of flour depends on the presence of flavonoids, anthocyanins, tannins and other pigments38.
Figure. 1. Plot of chromatic coordinates (a*- b*) of flours studied
The lightness (L) of the sample decreased in the following order: cassava (94.5) > maize (92.1) > taro (91.4) > banana (84.1). The cassava flour exhibited the highest L values, which may be attributed to factors such as the particle size of the flour, its composition, as well as other factors, including the variety of cassava and growing conditions. The reduction of the L value in the taro flour could be due to non-enzymatic browning reactions induced by drying the taro slices during the processing of taro tubers into flour18. On the other hand, banana flour presented the lowest L value, as reported by other authors, which could be related to enzymatic reactions. Hasmadi et al. (2020)32 report significantly different L values of 92.45 and 94.09 (significantly different) for cassava flours grown in other locations, attributing these variations to several factors, such as geographical location, variety, plant age and environmental conditions. The chromatic coordinates (a*(+a* = redness/-a*= greenness), b* (+b* = yellowness/-b*= blueness)) of the flours studied are also shown in Figure 1. The color of banana flour had the highest a* value (4.94), followed by maize flour (3.54), taro flour (2.77) and lastly, cassava flour. According to Figure 1, the cassava flour a* value (1 ± 0.1) was near zero, indicating no domination of red over green color. Values of b* parameter were much higher than a* for all studied flours except taro flour. Maize flour showed the highest b* values (27.4 ± 0.2), which is indicative of its yellow color due to the presence of a high amount of carotenoids22.
Hydration properties of samples
Water absorption capacity (WAC), water absorption index (WAI), water solubility index (WSI) and swelling power (SP) of taro, cassava, maize and banana flours are shown in Table 3. All hydration properties showed significant differences (p < 0.05) between samples. WAC values were maize (1.345 ± 0.006 g/g) > banana (1.224 ± 0.003 g/g) > cassava (1.167 ± 0.0.003 g/g) > taro (1.143 ± 0.003 g/g). WAC is associated with the number of hydrophilic groups present in proteins and carbohydrates in the flour39 and has a fundamental importance in viscous foods such as soups, sauces, noodles, doughs and baked products in which good protein-water interaction is required13,32 making flours with higher WAC to be a more suitable ingredient in gluten-free formulations. The WAI of different kinds of flours was in the range of 11.6 g/g to 6.4 g/g, whereas the highest value was observed for taro (11.60 ± 0.02 g/g) and the lowest value for banana (6.4 ± 0.1 g/g). The WAI values for all samples were significantly different (p < 0.05). WAI indicates the volume the starch granules occupy after swelling in excess water. WSI is related to the presence of soluble molecules that differed significantly among different flours29. WSI of other flours varied from 1.7 to 5.2 g/100 g (Table 3) and followed the order: taro < maize < cassava < banana. Lower solubility may be due to the lower amylose content, suggesting that the starch contained in taro flours possesses a low amylose ratio 41, following the values presented in section 3.1. They suggest that higher WSI flours such as banana and cassava can be used to increase the amount of food-soluble materials, such as starch and amino acids, which can be easily digestible29. Swelling power values of flours presented similar trends to WAI in the range of 11.8 g/g to 6.7 g/g and followed the order: taro (11.8 ± 0.02 g/g) > cassava (10.3 ± 0.4 g/g) > maize (8.02 ± 0.09 g/g) > banana (6.7 ± 0.1 g/g). SP has been related to the associative binding within the starch granules. The strength and character of the micellar network are related to the amylose content of the starch. Low amylose content produces high swelling power, which could explain the higher values of SP of taro and cassava flours. SP is a measure of hydration capacity, and when flour is heated above the gelatinization range in excess water, hydrogen bonds stabilizing the structure of the double helices in crystallites are disrupted. They are embraced with water, thus leading to flour swelling and increased overall volume41. The SP of taro and cassava flour is functionally beneficial in use as a thickener in the food industry and its solubility. Thickening is required in soups, gravies, baby foods, and breakfast gruels21. Variations in hydration properties between studied flours can be attributed to different origins, growing conditions of sources, and composition and amylose-amylopectin contents of starch granules.
Pasting behavior
The pasting property parameters of agro-residues (taro, cassava, maize and banana) flours are presented in Table 3 and RVA viscographs are shown in Figure 2. Flour’s pasting properties are essential in determining its suitability for application in the food industry. Maize flour presented the lowest pasting temperature (PT) values of 77.4 ᵒC, followed by taro and cassava, which gave slightly higher values (78.3 ± 0.1 ᵒC and 79 ± 1 ᵒC, respectively). Finally, the highest PT values were shown by banana flour (81.3 ± 0.5 ᵒC). More elevated PT in banana flour indicates higher thermal resistance of the starch to swelling and rupture14. The high PT could be due to non-starch components (i.e., proteins, oligosaccharides, cellulose). These compounds compete with the starch for water, reducing water availability and increasing the pasting temperature. Besides, the resistant starch in bananas contributes to a higher resistance to swelling and rupturing42. Thus, the lower the PT in flours, the weaker the intra-granular bonding forces and, therefore, less energy is required to damage the starch structure and paste formation, leading to economic advantages in saving energy costs. The analyzed flours presented a range for peak viscosity (PV) between 1266 – 3421 cP, where the banana flour presented the highest values, while the taro showed the lowest viscosity values. Chang presents similar results for PV in banana flour. et al. (2022)43. PV represented the maximum granule swelling point during heating and reflected the water absorption capacity of starch44. The higher PV of banana samples indicates the ability to form strong gel compared to other samples. This characteristic makes them potential for use as a thickener in products requiring sterilization, such as sauces and baby foods43. The breakdownbreakdown, defined as the numerical difference between the maximum (PV )and minimum viscosities (TV) at constant temperature (95 ᵒC), is associated with gel stability. Cassava and banana flours showed higher BV values,
Figure 2. Pasting profiles of samples analyzed. The discontinuous red line represents the temperature (right ordinate axis)
which indicates the higher swelling ability of starch and rapid re-association ability of leached amylose chain39. At the same time, lower BV suggested that the paste is more stable during cooking42. Low BV values in taro and maize flours indicate the potential of these flours to be used under high shear conditions during cooking13. Final viscosity (FV) shows the ability of the material to form a viscous paste, and the retrogradation of soluble amylose mainly determines it in the process of cooling13. Taro and cassava samples showed lower values of FV compared to banana and maize samples; values varied in the following order: banana (3859 ± 19 cP) > maize (3657 ± 37 cP) > cassava (1182 ± 4 cP) > taro (993 ± 6 cP). Therefore, flours of high FV, such as bananas and maize, may be helpful in bakery and confectionery products45. The setback oscillated between 191 and 2250 cP, where the maize flour presented the highest values and taro flour had the lowest values. Setback viscosity was related to the setback potential of starch, which reflected the stability of the starch paste during the cooling process. Low values of setback represent a slight tendency to retrograde42. Low amylose flours have low setback viscosity, and this suggests that amylose is essential to forming a three-dimensional network during cooling 46 following the amylose values presented in section 3.1 and confirms that taro flour retrograde to a lesser extent than other flours analyzed. Maize had the highest setback value. This characteristic indicated an increase in retrogradation tendency, which may provide the possibility for its application as a gel food formulation, such as Chinese straight rice vermicelli, rice noodles and sheet jelly43.
Steady shear rheological properties
Table 4. Steady shear properties of sample flours fitted to Herschel-Bulkley (σ = σo + k·γn).
The flow properties of sample flour dispersions were studied in the shear rate range 0.1 – 100 s-1, and the results are presented in Table 4. Fig 3. Show the flow curves (up and down) and the apparent viscosity (Pa·s) vs. shear rate (s-1) of analyzed samples. All the tested flour systems behaved to an excellent approximation (R2 = 0.995 – 0.999) as Bingham pseudoplastic with the Herschel-Buckley model over this range of shear rates. The yield stress (σo), or stress required to achieve the gel flow, represents the breakdown-breakdown of their structure under steady shear flow tests16. The σ0 values followed the order: maize (77 ± 4 Pa) > banana (43 ± 2 Pa) > taro (23 ± 1 Pa) > cassava (14 ± 1 Pa). Higher yield stress values confirm maize flours form stronger gels than banana, taro and cassava, indicating that it required higher force to initiate the flow of paste47. All samples showed threshold stress, suggesting that they exhibited solid behavior under low stress and liquid behavior under high stress, so the actual flow is only appreciable above a specific threshold value 48. k (consistency index) value of flour pastes varied from 4.8 to 23.3 Pa·s. Flour pastes from banana (23.3 ± 0.5 Pa·sn) and maize (19 ± 2 Pa·sn) had higher k values, which indicates its high structural strength in comparison to taro (5.3 ± 0.2 Pa·sn) and cassava (4.8 ± 0.2 Pa·sn) pastes. The low flow behavior index (n < 1) indicated that all the flours had a pseudoplastic, shear-thinning behavior, n ranked between 0.440 to 0.584, which meant that the rate of disruption of the intermolecular entanglements was more significant than the rate of reformation with the increase of shear rate, resulting in a reduction in apparent viscosity (Fig. 3 (b))49. The decrease in density with shear rate could be attributed to breaking the entanglement between the chains of the polymeric matrix, which induces a more random orientation of the molecules so that the interactions between adjacent chains are reduced, decreasing viscosity48. In addition, the «up» and «down» measurements showed a hysteresis loop, indicating that all samples are thixotropic. This is likely due to the shear inducing the break-up and homogenization of starch aggregates50.
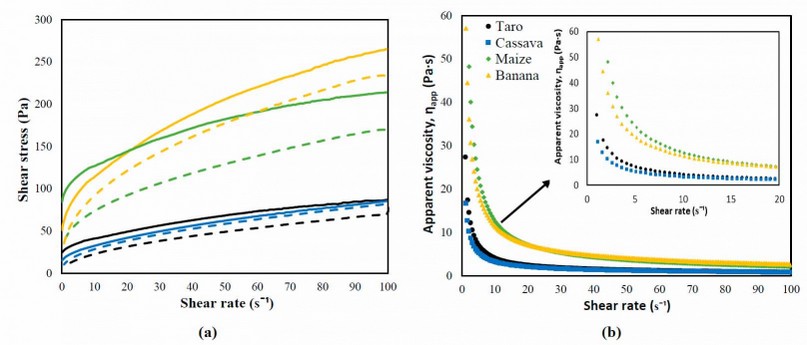
Figure 3. Steady shear flow behavior of 8% (w/w) samples pastes. a) Flow curves of taro (black lines), cassava (blue lines), maize (green lines) and banana (yellow lines) flours during 0.1 to 100 s-1 (solid lines) and 100 to 0.1 s-1 (dashed lines). b) Apparent viscosity (Pa·s) vs. Shear rate (s-1).
The largest hysteresis loop area was observed for the maize sample (Fig. 3 (a)), which seems most susceptible to shear time16. Bananas had a place two times smaller than maize and similar to taro. The smallest area was presented for cassava, indicating more excellent stability to shear time. Overall, threshold stress presented higher values in the upward sweep than in the downward sweep for all samples; moreover, during the upward sweep, the k values were much smaller than that in the low curves, indicating more shear-thinning behavior. Furthermore, the n values of the upward curve were larger than that of the downward curve, which showed more pseudo plasticity to shear time. In general, studying the rheological properties of these fluids makes it possible to establish and calculate transport and pumping systems and evaluate the behavior of gels when used as ingredients in fluid foods such as ketchup, mustard, and other types of sauces.
CONCLUSIONS
Crop residue flours showed nutritional, hydration and rheological properties, making them an attractive option for different food systems and industrial applications. The results revealed that studied characteristics of taro, cassava, maize and banana flours depended on several factors like the species, cultivars, variety, locality where it was harvested, cultivation practices, chemical composition of the soil, botanical origin, physiological state of the plant, and environmental growth conditions, etc. The flour made from maize showed higher yield and protein, fat and amylose content values than the other analyzed samples. All flours are good sources of carbohydrates, principally starch. The least gelation concentration of cassava and maize flours was higher than in the taro and banana samples. Maize flour showed the highest water absorption capacity values, while taro showed the highest values of water solubility index and swelling power between samples. Banana flour showed the highest pasting profile, while taro flour presented the lowest profile. The hysteresis loop area and yield stress of maize flour were significantly higher than all flours analyzed. The Banana samples showed the highest consistency index in the upward and downward curves, while the taro showed the highest flow behavior index. The results indicate that the flours studied offer new alternatives to maximize the benefits of crops affected by climate change, mainly because flours have a good potential of being used in food-processing fields as alternative ingredients.
Author Contributions: Caleb S. Calix-Rivera: Writing – original draft, Writing – review & editing, Formal analysis, conceptualization, methodology, conceived and designed the experiments; Analyzed and interpreted the data; Contributed analysis tools or data; Wrote the paper, performed the experiments. Rito J. Mendoza-Perez: Writing – review & editing, analyzed and interpreted the data and reviewed the paper. Octavio Rivera-Flores: Writing – review & editing, analyzed and interpreted the data and reviewed the paper. Felicidad Ronda: Funding acquisition, Resources, Investigation, Visualization, Supervision, Writing – review & editing, Project administration, Formal analysis, conceived and designed the experiments; Contributed reagents and materials.
Funding: The authors thank the financial support of the Ministerio de Ciencia e Innovación (PID2019-110809RBI00/AEI/10.1303/501100011033) and the Junta de Castilla y León/FEDER (VA195P20).
Informed Consent Statement: Not applicable
Acknowledgments: Authors thank the project Bioplástico EUROSAN / UNAH-TEC Danlí (Grant contract external actions of the European Union No. LA/2019/406-056) and the laboratory technicians Karla Martinez and Genesis Nohal Limas for their help in processing the flours analyzed. Caleb S. Calix-Rivera thanks the University of Valladolid for the doctorate grant. Rito J. Mendoza-Perez thanks the Junta de Castilla y León for the doctorate grant.
Conflicts of Interest: The authors declare no conflict of interest
REFERENCES
1. Alvi, T., Asif, Z. & Iqbal Khan, M. K. Clean label extraction of bioactive compounds from food waste through microwave-assisted extraction technique-A review. Food Biosci. 2022, 46.
2. Amicarelli, V. & Bux, C. Food waste measurement toward a fair, healthy and environmental-friendly food system: a critical review. British Food Journal 2020, 123 2907–2935.
3. FAO. 12.3.1 Global food losses | Sustainable Development Goals | Food and Agriculture Organization of the United Nations. Sustainable Development Goals https://www.fao.org/sustainable-development-goals/indicators/1231/en/ (2020).
4. Lopez Barrera, E. & Hertel, T. Global food waste across the income spectrum: Implications for food prices, production and resource use. Food Policy 2021, 98, 101874.
5. Kumar, S., Christakis, N. A. & Pérez-Escamilla, R. Household food insecurity and health in a high-migration area in rural Honduras. SSM – Popul. Heal. 2021, 15.
6. Lara-Arévalo, J., Escobar-Burgos, L., Moore, E. R. H., Neff, R. & Spiker, M. L. COVID-19, Climate Change, and Conflict in Honduras: A food system disruption analysis. Global Food Security 2023, 37 100693.
7. Palacios, H. V., Sexsmith, K., Matheu, M. & Gonzalez, A. R. Gendered adaptations to climate change in the Honduran coffee sector. Womens. Stud. Int. Forum 2023, 98, 102720.
8. USAID. Climate change risk profile: Honduras. USAID 1–5 https://www.climatelinks.org/sites/default/files/asset/document/2017_USAID ATLAS_Climate Change Risk Profile_Honduras.pdf (2017).
9. Segura-Badilla, O. Kammar-García, A. Mosso-Vázquez, J. Ávila-Sosa Sánchez, R. Ochoa-Velasco, C. Hernández-Carranza, P. Navarro-Cruz, A, R. Potential use of banana peel (Musa cavendish) as ingredient for pasta and bakery products. Heliyon 2022, 8, e11044.
10. AACC. Approved Methods of the American Association of Cereal Chemists. (AACC, 2000).
11. AOAC. Method 923.05-1923. in Official methods of analysis (Association of Official Analytical Chemists, 1996).
12. Singh, A., Sharma, S. & Singh, B. Influence of grain activation conditions on functional characteristics of brown rice flour. Food Sci. Technol. Int. 2017, 23, 500–512.
13. Abebe, W., Collar, C. & Ronda, F. Impact of variety type and particle size distribution on starch enzymatic hydrolysis and functional properties of tef flours. Carbohydr. Polym. 2015, 115, 260–268.
14. Calix-Rivera, C. S., Villanueva, M., Náthia-Neves, G. & Ronda, F. Changes on Techno-Functional, Thermal, Rheological, and Microstructural Properties of Tef Flours Induced by Microwave Radiation—Development of New Improved Gluten-Free Ingredients. Foods 2023, 12, 1345.
15. AACC. Method 76-21.02 General. in Approved Methods of the AACC (American Association of Cereal Chemists, 2009). doi:10.1094/AACCIntMethod-76-21.01.
16. Acevedo, B. A., Villanueva, M., Chaves, M. G., Avanza, M. V. & Ronda, F. Starch enzymatic hydrolysis, structural, thermal and rheological properties of pigeon pea (Cajanus cajan) and dolichos bean (Dolichos lab-lab) legume starches. Int. J. Food Sci. Technol. 2020, 55, 712–719.
17. Tambo Tene, S., Klang, J. M., Ndomou Houketchang, S. C., Teboukeu Boungo, G. & Womeni, H. M. Characterization of corn, cassava, and commercial flours: Use of amylase-rich flours of germinated corn and sweet potato in the reduction of the consistency of the gruels made from these flours—Influence on the nutritional and energy value. Food Sci. Nutr. 2019, 7, 1190–1206.
18. Rodríguez-Miranda, J. Ruiz-López, I. I. Herman-Lara, E. Martínez-Sánchez, C. E. Delgado-Licon, E. Vivar-Vera, M. A. Development of extruded snacks using taro (Colocasia esculenta) and nixtamalized maize (Zea mays) flour blends. Lwt 2011, 44, 673–680.
19. Abioye, V. F., O Ade-Omowaye, B. I., Babarinde, G. O. & Adesigbin, M. K. Chemical, physico-chemical and sensory properties of soy-plantain flour. African J. Food Sci. 2011, 5, 176–180.
20. Mbofung, C. M. F., Aboubakar, Y. N., Njintang, A., Abdou Bouba, A. & Balaam, F. Physicochemical and Functional Properties of Six Varieties of Taro (Colocasia esculenta L. Schott) Flour. J. Food Technol. 2006, 4, 135–142.
21. Ayetigbo, O., Latif, S., Abass, A. & Müller, J. Comparing characteristics of root, flour and starch of biofortified yellow-flesh and white-flesh cassava variants, and sustainability considerations: A review. Sustainability. 2018, 10, 3089.
22. Moreira, R., Chen, F., Arufe, S. & Rubinos, S. N. Physicochemical characterization of white, yellow and purple maize flours and rheological characterization of their doughs. J. Food Sci. Technol. 2015, 52, 7954–7963.
23. Campuzano, A., Rosell, C. M. & Cornejo, F. Physicochemical and nutritional characteristics of banana flour during ripening. Food Chem. 2018, 256, 11–17.
24. Ai, Y. & Jane, J. Understanding Starch Structure and Functionality. in Starch in Food 2018, 151–178. doi:10.1016/B978-0-08-100868-3.00003-2.
25. Hoover, R. Composition, molecular structure, and physicochemical properties of tuber and root starches: A review. Carbohydr. Polym. 2001, 45, 253–267.
26. Oyeyinka, S. A., Adeloye, A. A., Smith, S. A., Adesina, B. O. & Akinwande, F. F. Physicochemical properties of flour and starch from two cassava varieties. Agrosearch 2019, 19, 28.
27. Hoover, R., Hughes, T., Chung, H. J. & Liu, Q. Composition, molecular structure, properties, and modification of pulse starches: A review. Food Res. Int. 2010, 43, 399–413.
28. Olawoye, B., Fagbohun, O. F., Popoola, O. O., Gbadamosi, S. O. & Akanbi, C. T. Understanding how different modification processes affect the physiochemical, functional, thermal, morphological structures and digestibility of cardaba banana starch. Int. J. Biol. Macromol. 2022, 201, 158–172.
29. Kaushal, P., Kumar, V. & Sharma, H. K. Comparative study of physicochemical, functional, antinutritional and pasting properties of taro (Colocasia esculenta), rice (Oryza sativa) flour, pigeonpea (Cajanus cajan) flour and their blends. LWT – Food Sci. Technol. 2012, 48, 59–68.
30. Tagodoe, A. & Nip, W. ‐K. Functional properties of raw and precooked taro (Colocasia esculenta) flours. Int. J. Food Sci. Technol. 1994, 29, 457–462.
31. Solaesa, Á. G., Villanueva, M., Vela, A. J. & Ronda, F. Protein and lipid enrichment of quinoa (cv.Titicaca) by dry fractionation. Techno-functional, thermal and rheological properties of milling fractions. Food Hydrocoll. 2020, 105, 105770.
32. Hasmadi, M. Harlina, L. Jau-Shya, L. Mansoor, A. H. Jahurul, M. H.A. Zainol, M. K.. Physicochemical and functional properties of cassava flour grown in different locations in Sabah, Malaysia. Food Res. 2020, 4, 991–999.
33. Bolade, M. K., Adeyemi, I. A. & Ogunsua, A. O. Influence of particle size fractions on the physicochemical properties of maize flour and textural characteristics of a maize-based nonfermented food gel. Int. J. Food Sci. Technol. 2009, 44, 646–655.
34. Pragati, S., I, G. & Ravish, K. Comparative Study of Ripe and Unripe Banana Flour during Storage. J. Food Process. Technol. 2014, 5.
35. Marcel, N. R., Patrick, Y., Seraphine, E. & Robert, N. Utilization of overripe banana/plantain-maize composite flours for making doughnuts: physicochemical, functional, rheological and sensory characterization. J. Food Meas. Charact. 2021, 15, 59–70.
36. Rafiq, S. I., Singh, S. & Saxena, D. C. Physical, physicochemical and anti-nutritional properties of Horse Chestnut (Aesculus indica) seed. J. Food Meas. Charact. 2016, 10, 302–310 (2016).
37. Eke-Ejiofor, J. & Friday, U. B. Physicochemical, functional and pasting properties of starch from breadfruit (Artocarpus altilis) in gurudi snack production. J. Biotechnol. 2019, 7, 38–48.
38. Kumar, S. R., Sadiq, M. B. & Anal, A. K. Comparative study of physicochemical and functional properties of pan and microwave cooked underutilized millets (proso and little). LWT 2020, 128.
39. Dhua, S. Kheto, A. Sharanagat, V. Singh, L. Kumar, K. Prabhat K. Quality characteristics of sand, pan and microwave roasted pigmented wheat (Triticum aestivum). Food Chem. 2021, 365, 130372.
40. Kumar, V., Sharma, H. K. & Singh, K. Effect of precooking on drying kinetics of taro (Colocasia esculenta) slices and quality of its flours. Food Biosci. 2017, 20, 178–186.
41. Huang, S., Martinez, M. M. & Bohrer, B. M. The compositional and functional attributes of commercial flours from tropical fruits (breadfruit and banana). Foods 2019, 8, 586.
42. Bento, J. A. C. Morais, D. de Berse, R. Bassinello, P. Caliari, M. Soares J, M. Functional, thermal, and pasting properties of cooked carioca bean (Phaseolus vulgaris L.) flours. Appl. Food Res. 2022, 2.
43. Chang, L. Yang, M. Zhao, N. Xie, F. Zheng, P. Simbo, J. Yu, X. Du, S. Structural, physicochemical, antioxidant and in vitro digestibility properties of banana flours from different banana varieties (Musa spp.). Food Biosci. 2022, 47, 101624.
44. Zhou, Y. Wang, M. Li, W. Liu, G. Wang, W. Zhi, W. Wang, M. Wang, R. Hu, A. Zheng, J. Effects of dual modification of lysine and microwave on corn starch: In vitro digestibility and physicochemical properties. Int. J. Biol. Macromol. 2022, 220, 426–434.
45. Ayo-Omogie, H. N., Jolayemi, O. S. & Chinma, C. E. Fermentation and blanching as adaptable strategies to improve nutritional and functional properties of unripe Cardaba banana flour. J. Agric. Food Res. 2021, 6, 100214.
46. Emmambux, M. N. & Taylor, J. R. N. Morphology, physical, chemical, and functional properties of starches from cereals, legumes, and tubers cultivated in Africa: A review. Starch – Stärke 2013, 65, 715–729.
47. Sandhu, K. S., Siroha, A. K., Punia, S. & Nehra, M. Effect of heat moisture treatment on rheological and in vitro digestibility properties of pearl millet starches. Carbohydr. Polym. Technol. Appl. 2020, 1, 100002.
48. Salazar, D. Arancibia, M. Lalaleo, D. Rodríguez-Maecker, R. López-Caballero, M. Montero, M. . Physico-chemical properties and filmogenic aptitude for edible packaging of Ecuadorian discard green banana flours (Musa acuminanta AAA). Food Hydrocoll. 2021, 122.
49. Chen, X. Liu, Y. Xu, Z. Zhang, C. Liu, X. Sui, Z. Corke, H. Microwave irradiation alters the rheological properties and molecular structure of hull-less barley starch. Food Hydrocoll. 2021, 120, 106821.
50. Kang, N., Zuo, Y. J., Hilliou, L., Ashokkumar, M. & Hemar, Y. Viscosity and hydrodynamic radius relationship of high-power ultrasound depolymerized starch pastes with different amylose content. Food Hydrocoll. 2016, 52, 183–191.
Received: 20 June 2023/ Accepted: 25 August 2023 / Published:15 September 2023
Citation: Calix-Rivera C S, Mendoza-Perez R J, Rivera-Flores O, Ronda F. Physicochemical, Hydration and Steady Shear Rheological Properties of Flours Derived From Different Crop Residues From the Honduran Agro–Food Industry.Revis Bionatura 2023;8 (3) 34. http://dx.doi.org/10.21931/RB/2023.08.03.34