Vol 8 No 2 2023 – 18
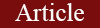
Coordination compounds of carbonyl oxygen polychelate ligand; synthesis, physicochemical characterization and biological evaluation
1Department of Chemistry, College of Education for Pure Science (Ibn Al-Haitham), University of Baghdad, Adhamiyah, Baghdad, Iraq
*Corresponding author; Emails: mohamad.al-jeboori@ihcoedu.uobaghdad.edu.iq;
mohamadaljeboori@yahoo.com
Available from: http://dx.doi.org/10.21931/RB/2023.08.02.18
ABSTRACT
The work covers the formation of the bisaldehyde ligand 2,2′-[ethane-1,2diylbis(oxy)] dibenzaldehyde (M) and its metal complexes. The synthesis of M was achieved via two steps in which two equivalents of the salicylaldehyde were reacted with KOH in an ethanolic medium. Subsequently, the resulting compound dissolved in DMF and one equivalent of 1,2-dibromoethane was added. Adding an ethanolic solution of metal chloride of a range of metal ions to the ligand in a 1:1 (M:L) mole ratio resulted in preparing a new five monomeric complexes. The entity of the isolated structure for the prepared compounds was confirmed using physicochemical methods (FT-IR, electronic spectroscopy, mass and 1H, 13C-NMR spectra, elemental microanalysis, magnetic susceptibility and molar conductance). The characterization data indicated the isolation of six and four-coordinate monomeric complexes with the general formula; [Cr(M)(Cl)2(H2O)2]Cl, [M(M)(Cl)2].H2O (where M= Fe(II), Co(II) and Cu(II)) and [Ni(M)]Cl2.H2O. The anti-microbiological activity of the title compounds (ligand and complexes) was tested against various bacterial and fungal microorganisms. The acquired data revealed that the metal complexes became potentially more active than the M.
Keywords: Bisaldehyde ligand; Novel monomeric metal complexes; Structural study; Antimicrobial activity.
INTRODUCTION
The current interests in forming bis-functionalized compounds are due to their wide range of uses in organic and inorganic chemistry, biology and industry 1,2. These materials are fascinating precursors employed as building blocks in supramolecule and nanotechnology 2,3. Aromatic aldehydes are natural product species classified as essential compounds due to their uses in the fragrance and cosmetics industries, food technology, medicine, and pharmaceuticals 4,5. Further, the condensation reaction of these species, the bisaldehyde compounds, with a primary amine segment resulted in the preparation of the Schiff base. Compounds that are derived from bis-functionalized starting materials, in particular, 2,2′-[ethane-1,2diylbis(oxy)]dibenzaldehyde (EDD) precursor, are interesting organic species that are used in the formation of Schiff-bases. The formation of the EDD is based on the reaction of the dibromoalkyl with the salicylaldehyde in the presence of an alkali medium. Several condition reactions were implemented to form the EDD precursor to optimize yield, solvent and other reaction factors; schiff bases derived from the EDD were used as complexation agents and a range of metal complexes were reported 6. Recently, we reported the isolation of complexes derived from Mannich bases in which carbonyl oxygen moiety of the cyclohexanone segment is involved in coordinating with the metal centers 7,8. However, to our knowledge, there are no published reports on using EDD as a complexation agent. Therefore, the current work intends to explore the potential ability of EDD as a complexation agent in which carbonyl oxygen of the bisaldehyde moieties is involved in the coordination with the metal center. The preparation of 2,2′-[ethane-1,2diylbis(oxy)]dibenzaldehyde (EDD) with a modification procedure to that reported previously 9 and the formation of a range of monomeric complexes derived from EDD are reported. Further, this study explores the complexation effect on the antimicrobial activity on the free bisaldehyde ligand upon coordination with the mental center.
MATERIALS AND METHODS
The organic and inorganic materials used in this work were commercially available and were used as received. The FTIR spectra of compounds were recorded using KBr and CsI discs from 4000–250 cm-1 on a Shimadzu Fourier Transform Infrared Spectrometer (FTIR-600). The mass spectrum for the ligand was determined using the electrospray method (positive mode) with the Agilent mass spectrometer Sciex ESI mass analysis. The ligand’s 1H- and 13C-NMR spectra were recorded in DMSO-d6 with a Brucker-500 MHz. For the 1H-NMR study, tetramethylsilane (TMS) was used as an internal standard to measure chemical shifts. A Shimadzu UV-160 spectrophotometer was used to analyze the electronic spectra of compounds from 200–1000 nm for 10-3 M solutions in DMSO at ambient temperature. An electrothermal Stuart apparatus, model SMP40, was used to determine melting points. Elemental analyses (CHN) for the ligand and their metal complexes were performed using EuroEA 3000 machine. A Shimadzu (AA) 680G atomic absorption spectrophotometer and a potentiometric titration technique on a 686-nitro processor-665 Dosimat-Metrom Swiss were used to determine metal and chloride percentages for compounds, respectively. The magnetic moments were performed on a Sherwood Scientific Devised at 308 K. A Eutech Instruments Cyberscan con 510 digital conductivity meter was used to measure molar conductance for complexes.
Synthesis
Synthesis of 2,2′-(Ethane-1,2-diylbis(oxy))dibenzaldehyde (M)
The formation of M was based on a published method 9 with a modification and as follows: To an ethanolic solution of KOH (0.56g, 10mmol) in 15ml of absolute EtOH was added with stirring a mixture of salicylaldehyde (1.22g, 10mmol) in EtOH (5ml). The reaction mixture was allowed to reflux until a clear solution was obtained, and then the solvent was removed under reduced pressure to give a pale green solid. 10ml of DMF was added to the solid, and the mixture was heated at 100°C for 10min. Subsequently, a mixture of 1,2-dibromoethane (0.94g, 5 mmol) in DMF (5 ml) was added with stirring. The resulting mixture was allowed to reflux for 30min and stirred at room temperature, then distilled water was added. The mixture was allowed to stir for 2h, and the formed brown solid was collected by filtration and washed with a copious amount of water. The compound was recrystallized from hot ethanol, and bright brown crystals were collected by filtration that was kept in the air to dry. Yield: 2.4g (88%), m.p = 118 – 120°C. M.wt=270 amu. FT-IR (KBr) cm-1; 1685 and 1666 ν(C=O)ald, 1242 ν(C-O-C) and 1597 ν(C=C)cm-1.
Synthesis of complexes
The metal complexes were prepared following an analog procedure. Therefore, the formation of [Cr(M)(Cl)2(H2O)2]Cl is reported as a model for the preparation of complexes and as follows: To a stirring solution of the bisaldehyde ligand (0.10g, 0.37mmol) in 10ml of a hot mixture of DMF and ethanol with an 8:2 (v:v) ratio has added a solution of chromium(III) chloride hexahydrate (0.10g, 0.37mmol) dropwise in 10ml of ethanol. The resulting mixture was heated by stirring at 70℃ for 4h. The brownish-red solid that formed was collected by filtration, washed with diethyl ether (5ml) and dried in air. Yield: 0.122g (76%), m.p = 250-248℃. Table 1 includes elemental analytical data, colors, and products of complexes. Tables 2 and 3 show the FT-IR and electronic data of complexes, respectively.
Biological activity measurement
The evaluation of ligand and its metal complexes against four bacterial species (Escherichia coli, Pseudomonas aeruginosa, Staphylococcus aureus and Bacillus subtilis) and two types of fungi (Candida albicaus and Rhizopus sporium) were performed using agar-well diffusion 10. In this method, the wells were dug in the media with the help of a sterile metallic borer with centers of a diameter of at least 6 mm. The recommended concentration (100 μL) of the 1 mg/mL test sample in DMSO was introduced in the respective wells. The plates were incubated immediately at 37°C for 24h. The activity was evaluated by measuring the diameter of inhibition zones (mm). The samples were performed at the Central Service Laboratory / Ministry of Science and Technology / Baghdad / Iraq.
RESULTS AND DISCUSSION
The synthesis of the ligand (M), 2,2′-[ethane-1,2diylbis(oxy)] dibenzaldehyde, and its metal complexes are reported. The ligand was prepared with modification in a two-step. These include the reaction of salicylaldehyde with KOH in an ethanolic medium. After removing the solvent under vacuum, 1,2-dibromoethane in DMF medium was added and allowed to heat at reflux for 2h. The reaction mixture was allowed to stir at RT, and a precipitate was formed by adding distilled water. The title compound was collected by filtration and then recrystallized from EtOH to give M an excellent yield, Figure 1. The ligand (M) is a potentially tetradentate species that consist of two carbonyl oxygen-aldehydes and two oxygen-ether donor atoms. The reaction of M with metal chlorides of Cr(III), Fe(II), Co(II), Ni(II) and Cu(II) in a 1:1 (L:M) mole ratio using a mixture of EtOH/DMF in a 2:8 ratio at reflux gave the required complexes, Figure 2. The complexation reaction is solvent and temperature-dependent, and unidentified residues were obtained upon using different solvents or temperatures. The obtained data indicated the formation of six-coordinate monomeric complexes (bar [Ni(M)]Cl2.H2O that gave a four-coordinate monomeric complex). A variety of physicochemical tools were used to characterize the isolated compounds (ligand and complexes). These include; 1H, 13C-NMR and mass spectroscopy (for ligand), micro-elemental studies and metal and chloride ratio (Table 1), FT-IR (Table 2) and UV-Vis spectroscopy (Table 3). The isolation of electrolyte complexes ([Cr(M)(Cl)2(H2O)2]Cl and [Ni(M)]Cl2.H2O) and nonelectrolyte complexes of the general formula [M(M)(Cl)2].H2O (where M= Fe(II), Co(II) and Cu(II)) was determined by measuring their conductance in DMSO solutions, Table 3.
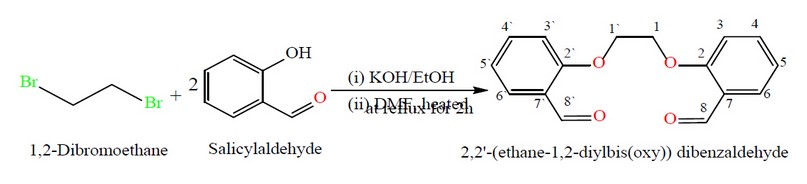
Figure 1. Preparation route and reaction conditions of the bisaldehyde ligand (M).
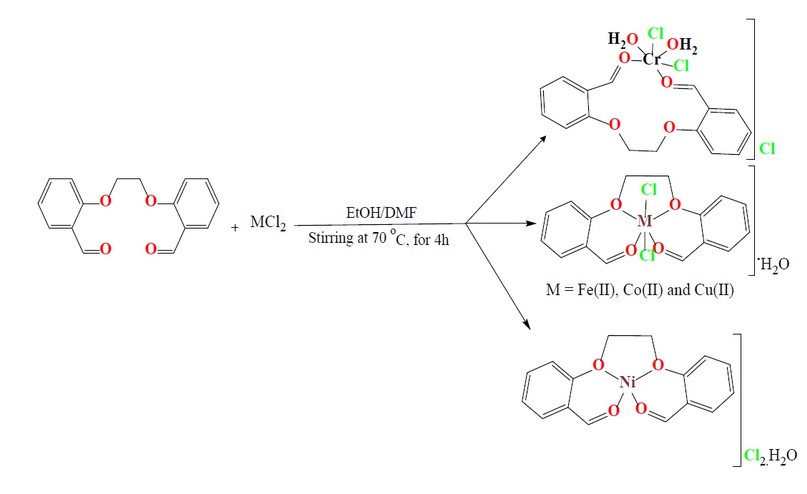
Figure 2. Synthesis route of monomeric complexes.
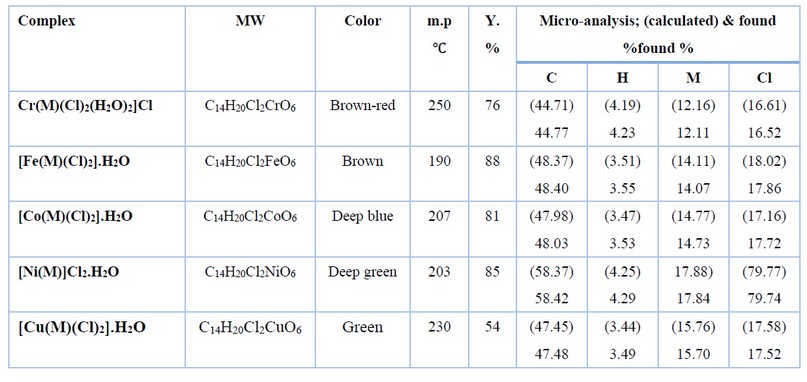
Table 1. The physical properties and elemental analyses of M and its complexes.
FT-IR data of bisaldehyde
The FT-IR spectrum of M, Figure 3, indicated two bands at 1685 and 1666 cm-1, which are due to the ν(C=O) group, compared with that at 1695 cm-1 for the ν(C=O) group of the salicylaldehyde. The appearance of two peaks indicates the two carbonyl groups are non-equivalent. The spectrum showed no peak around ca. 3200 and 600 cm-1 may be attributed to the ν(OH) and ν(C-Br) groups11 indicating the involvement of the O-H and the C-Br in the formation of the ether group. This was confirmed by the appearance of a band at 1242.16 cm-1 assigned to ν(C-O-C) stretching of the ether group 11,12.
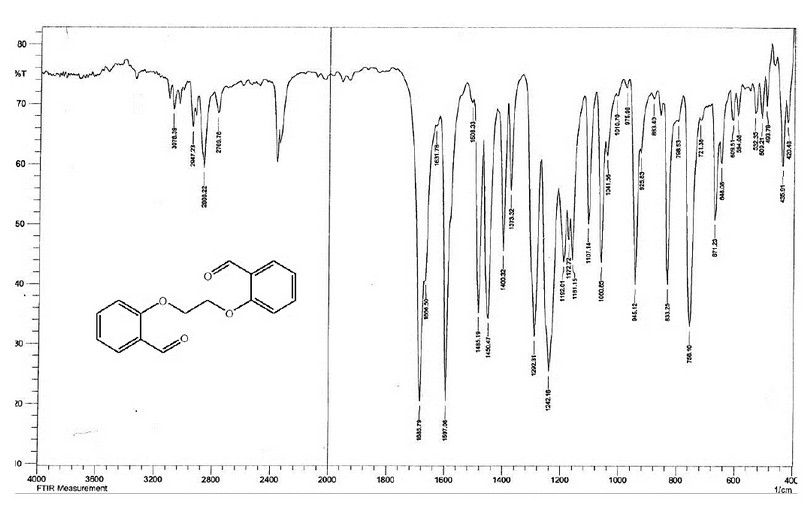
Figure 3. The FT-IR spectrum of the bisaldehyde ligand (M).
NMR and mass spectra of the bisaldehyde ligand
The 1H- and 13C-NMR of M were acquired in DMSO-d6 on a Brucker-500 MHz machine. The assignment of peaks is based on the numbering fashion reported in Figure 1). The 1H NMR spectrum of 2,2′-(ethane-1,2diylbis(oxy))dibenzaldehyde with an expansion of the aromatic region is shown in Figure 4. The spectrum showed several signals between 10.29-7.08 ppm in the aromatic region. The singlet peak at 10.29 ppm, equivalent to two protons, is related to (2H, s, C8,8`–H). The multiple chemical shift at 7.68-7.65 ppm is equivalent to four protons attributed to (4H, m, C6,6; 4,4`-H). A doublet peak at 7.34-7.32 ppm is equivalent to two protons attributed to (2H, d, C3,3,-H J = 8.2 Hz). The triplet peak at 7.11-7.08 ppm that equivalent to two protons assigned to (2H, t, C5,5`–H `, J = 7.45, 7.50 Hz). The chemical shift related to the ethylene group was detected as a singlet at 4.58 ppm (4H, s, H1,1`). The spectrum recorded signals at ca. 2.50 and 3.33 ppm related to the DMSO-d6 and the traces of water in the NMR solvent, respectively. The 13C NMR spectrum of M in the DMSO-d6 solution is placed in Figure 5. The spectrum indicated the correct resonances for the compound. Resonance related to the carbonyl group assigned to C8,8– appeared as a two signal at δc = 188.70 and 188.68 ppm. The appearance of two signals indicated that the two C=O groups are slightly inequivalent in solution. This follows the FT-IR data, Figure 3, in which the two carbonyl groups are also inequivalent in the solid state. Signal related to C2,2` appeared at δc = 160.39 ppm. Resonances correlated to C4,4`, C6,6– C5,5– C7,7– and C3,3– were observed at 135.94, 127.13, 124.11, 120.70 and 113.76 ppm, respectively. The two methylene groups, O-C1,1–, appeared to be equivalent and were detected at 67.00 ppm.
The electrospray (+) mass spectrum of M, Figure 6, reveals the parent ion peak at m/z=270.1 amu (18.22%). This peak is related to (M)+, calculated at 270.09 amu for C16H14O4. Peaks detected at m/z = 240.2 (4.52%), 197.1 (7.56%), 149.2 (70.55%),121.2 (100.0%), 105.1 (16.20%), 77.2 (48.10%) and 65.2 (40.56%) related to [M-(C15H12O3)]+, [M-(C13H9O2)]+, [M-(C9H9O2)]+, [M-(C7H5O2)]+, [M-(C7H5O)]+, [M-(C6H5)]+ and [M-(C5H5)]+, respectively. The assignment of the successive fragmentation ions of the compound, along with their relative abundance, is shown in Figure 7.
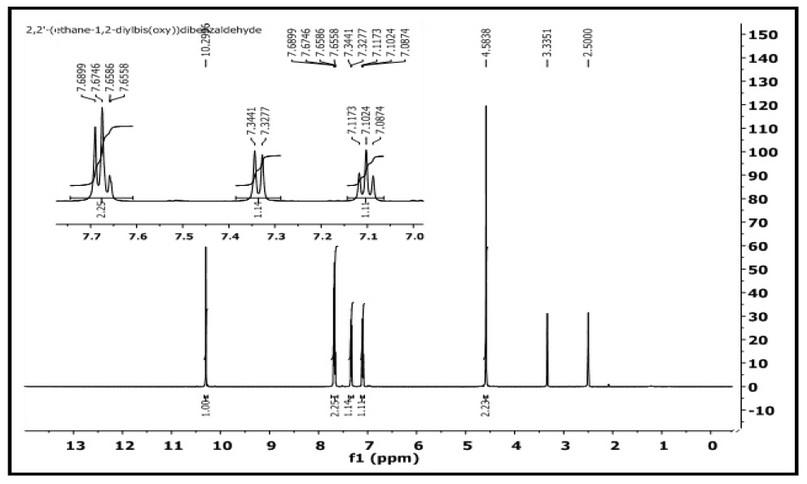
Figure 4. 1H-NMR spectrum of M in DMSO-d6.
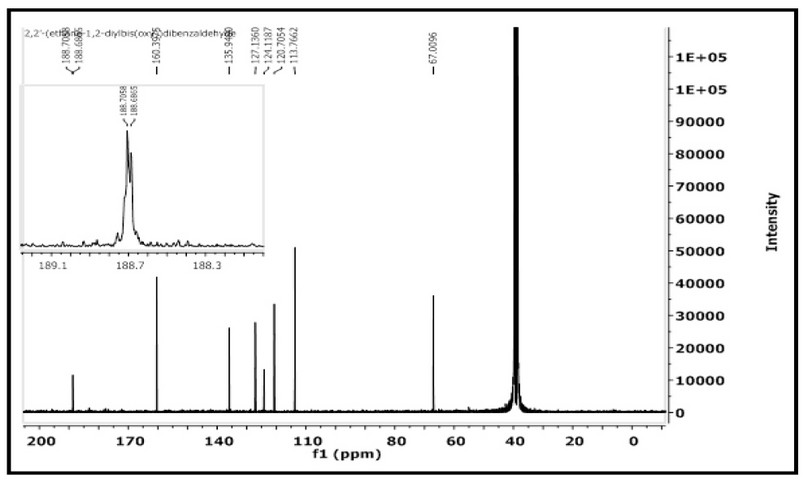
Figure 5. 13C-NMR spectrum of M in DMSO-d6 solution.
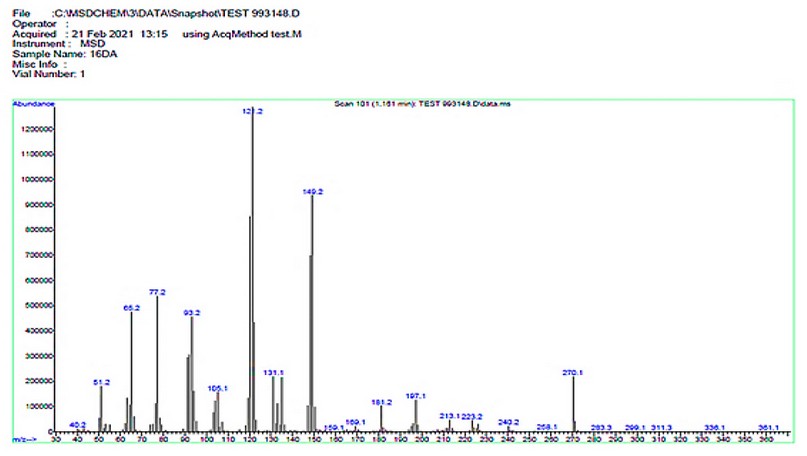
Figure 6. The electrospray (+) mass spectrum of M.
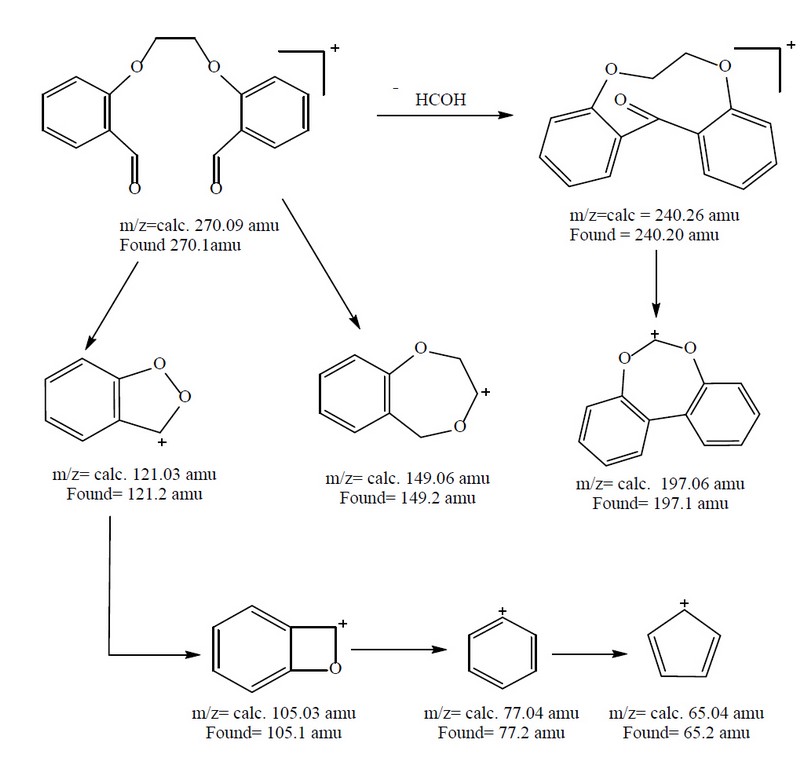
Figure 7. The fragmentation pattern and relative abundance of M.
FT- IR spectra of complexes
The FT-IR spectral data of the distinct bands for 1, 2, 3, 4, and 5 are placed in Table 2. The spectra of complexes were compared with the spectrum of the ligand (M) which indicated the involvement of the oxygen moieties, carbonyl oxygen-aldehydes and/or oxygen-ethers in coordination with the metal center. Bands related to the ν(C=O) of the carbonyl have suffered a higher shift in complex 4 and appeared at 1687 and 1670 cm-1, compared with that of M at 1685 and 1666 cm-1. However, this band was shifted by 4-47 cm-1 to a lower frequency in complexes 1, 2, 3, 4 and 5 and appeared at 1680;1654, 1651;1612, 1685;1662, 1687;1670 and 1643 cm-1, respectively. The shift of the carbonyl bands is related to the involvement of these moieties in the coordination with the metal center 12,13. Further, the spectra showed the two coordinated carbonyl groups are non-equivalent (except that for the Cu(II)-complex). The ν(C-O-C) ether of the M that was detected at 1242 cm-1 has shifted to a higher wavenumber by 4-54 cm-1 and appeared at 1253, 1253 and 1249 cm-1 in complexes 2, 4 and 5, respectively 11,14. However, in complex 3, the ether band was moved to a lower wavenumber and appeared at 1238 cm-1. More, in complex 1 there was no change in the frequency of the ν(C-O-C) ether group, which confirms the chromium(III) ion was not bound to oxygen ether. The shifting of the oxygen ether segment may contribute to the oxygen atoms’ involvement in the coordination with the metal center 11,14. The spectra of metal complexes revealed additional bands between 600-200 cm-1 that were not presented in the M spectrum. Bands that are related to ν(M-O) carbonyl were detected at 532, 520, 509, 532 and 509 cm-1 for ν(Cr-O), ν(Fe-O), ν(Co-O), ν(Ni-O) and ν(Cu-O), respectively 15,19,20. The ν(M-O) for the oxygen ether bands related to ν(Fe-O), ν(Co-O), ν(Ni-O) and ν(Cu-O) were detected at 574, 532, 582 and 578 cm-1, respectively 11,15. The FT-IR spectra revealed bands that belong to ν(Cr-Cl), ν(Fe-Cl), ν(Co-Cl) and ν(Cu-Cl) at 243;252, 241;248, 262;283 and 235;246 cm-1, respectively 16. Finally, peaks were detected at 3321, 3491, 3444, 3329 and 3471 cm-1 in the complexes of Cr(III), Fe(II), Co(II), Ni(II) and Cu(II), respectively, were correlated to aqua water molecules. In complex 1, a band that was detected at 756 cm-1 is related to ν(Cr-O) 17.
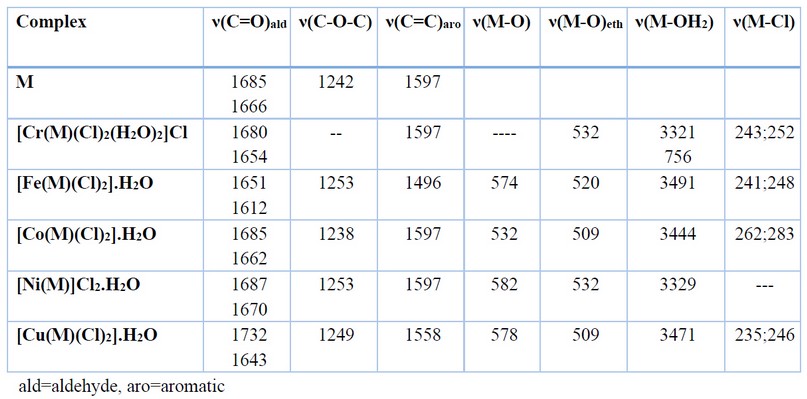
Table 2. The Infrared spectrum of the complexes prepared from (M).
Electronic spectra and magnetic moment measurements
The electronic absorption spectra of M, Figure 8, and its complexes were determined in DMSO solutions (con. = 1 x 10-3 M). The UV-Vis spectra of Cr(III), Fe(II), Co(II), Ni(II) and Cu(II) complexes indicated peaks around 263-265 nm related to π→π* and n→π* (ligand field transitions), Table 3. The spectra of 1, 2, 3, 4 and 5 showed an extra peak of about ca.315 nm assigned to charge transfer (CT) 16-18. In the d-d region of the Cr(III) complex (Figure 9), bands at 435 and 633 nm correlated to 4A2g(F)→4T1g and 4A2g(F)→4T2g(F), respectively indicating a distorted octahedral geometry about the metal center 19. The Fe(Ⅱ) complex revealed peaks, at 833, 889 and 977 nm correlated to 5T2g→2Eg, indicating an octahedral shape around the core atom 20. Peaks at 615 and 677 nm in the spectrum of Co(II) are referred to 4T1g(F) → 4A2g(F) and 4T1g(F) → 4A2g(F), respectively confirming a distorted octahedral geometry around the metal center 21,22. In the Ni(II)complex (Figure 10), peaks at 619, 652 and 692 nm attributed to 3T1(F)→ 3A2(F), 3T1(F)→ 3A2(F) and 3T1(F)→ 3T1(P) that supported a distorted tetrahedral geometry around the metal center 23. The broad peak at 645 nm in the spectrum of Cu(II) is referred to 2B1g→ 2B2g confirming a distorted octahedral geometry around the metal center 24. The magnetic value is an essential factor for the characterisation of complexes, as it may be linked to the shape adopted by ligands around the metal center. For Cr(III), Fe(II), Co(II), Ni(II) and Cu(II) complexes, the obtained magnetic moment was determined to be 3.74, 4.22, 4.35, 4.55 and 2.16 BM, respectively. These values matched the total of the spin’s moment, indicating a weak field with sp3d2 and sp3 hybridization of complexes. Therefore, Cr(III), Fe(II), Co(II) and Cu(II) complexes adopted a distorted octahedral geometry. However, the Ni(II) complex adopts a tetrahedral 24.
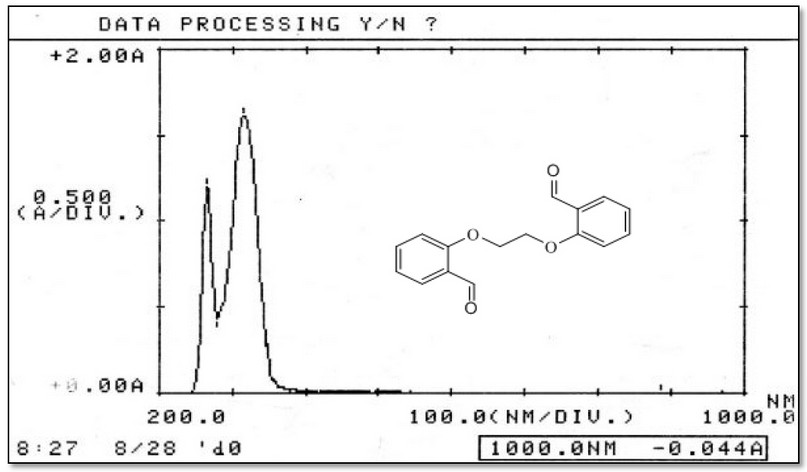
Figure 8. Electronic spectrum of the ligand (M) in DMSO solvent.
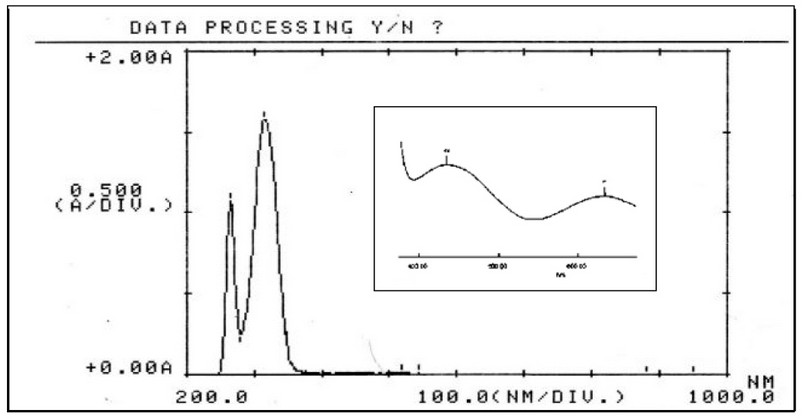
Figure 9. Electronic spectrum of [Cr(M)(Cl)2(H2O)2]Cl in DMSO solvent.
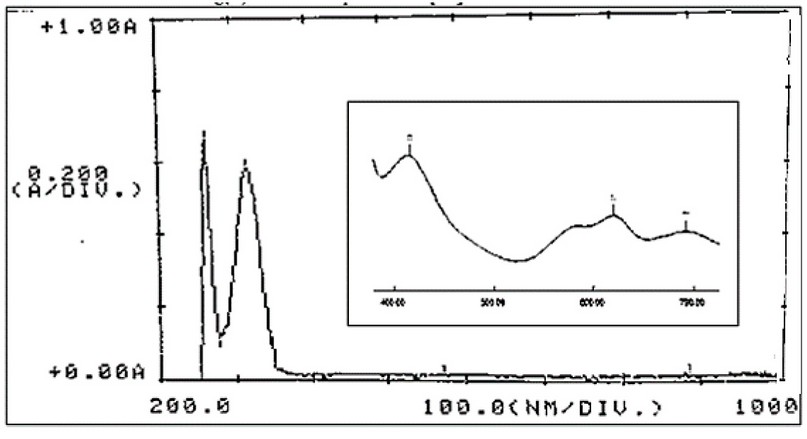
Figure 10. Electronic spectrum of [Ni(M)]Cl2.H2O in DMSO solvent.
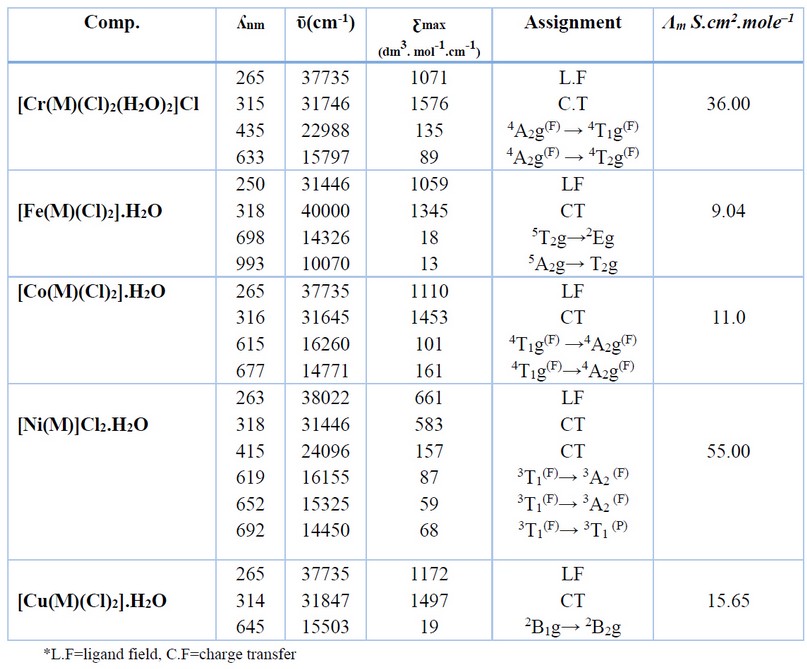
Table 3. Electronic spectral data and molar conductance for M complexes.
Biological activity
The prepared ligand and its metal complexes with Cr(III), Fe(II), Co(II), Ni(II) and Cu(II) ions were tested against two microbial strains; G-positive (Staphylococcus aureus, Bacillus subtilis), as well as G-negative (Escherichia coli, Pseudomonas auroginosa). The examined bacteria in this study infect humans’ urinary and respiratory tracts, and they are among the most dangerous and lethal pathogens discovered in surgical operating rooms. Further, two types of fungus that affect humans were investigated (Candida albicans and Rhizopus sporium) 8,17. The collected data is presented in Figure 11 and Table 4. According to the obtained results, the title compounds exhibit varied antibacterial action against microorganisms. The zone of inhibition ability of compounds was compared with Cefotaxime (100 μg/mL) used as an antibacterial standard. The following essential points are drawn from the gathered data that is given in the Tables:
1- All complexes showed activity against positive and negative bacteria.
2- Experimental data indicated the complexes became more active compared with M. Further, the prepared compounds indicated higher activity compared with cefotaxime.
3- Based on the experimental information, iron and cobalt complexes show higher microbiological activity against strains of (Escherichia coli) bacterial, whereas the nickel complex is less effective against (Escherichia coli). This may attribute to the influence of the cationic nature and the tetrahedral geometry of the nickel complex, compared with the neutral six-coordinate ion and cobalt complexes.
The antifungal activity of M and its metal complexes were tested against two types of fungi. The role of DMSO in the biological screening was concluded separately using the solutions of DMSO alone, which showed no activity towards fungal species 8,17. Fungi activity data against tested compounds are shown in Figure 12 and Table 4 and placed in. The following conclusions have been pointed out;
1- The M and its complexes generally showed good results against the rhizopus sporium, compared with fungi Candida albicans.
2- The Cr(III), Co(II) and Ni(II) -complex indicated the highest inhibition activity against Rhizopus sporium.
3- 3- Complexes of Cr(III) and Cu(II)) showed the lowest anti-fungal activity against Candida albicans.
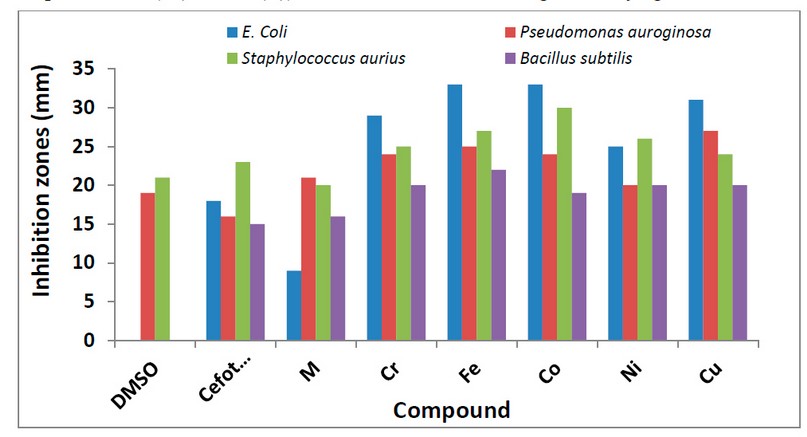
Figure 11. The inhibition zone diameter (mm) against bacterial species for M ligand and its complexes.
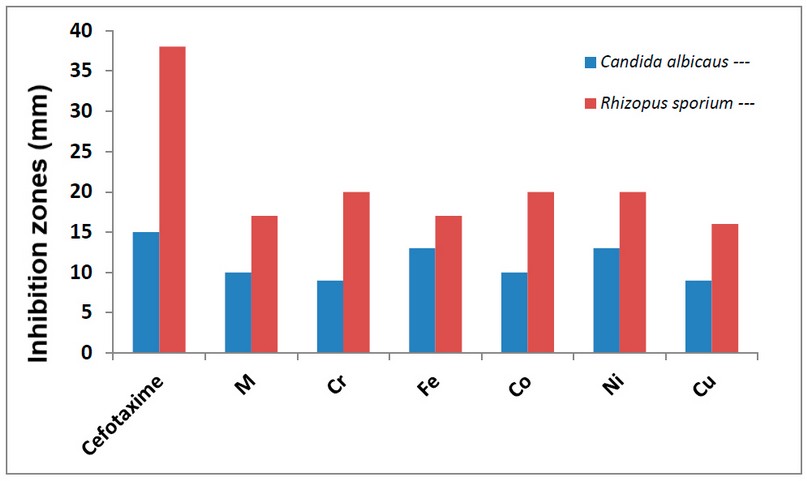
Figure 12. The inhibition zone diameter (mm) against fungi for M ligand and its complexes.
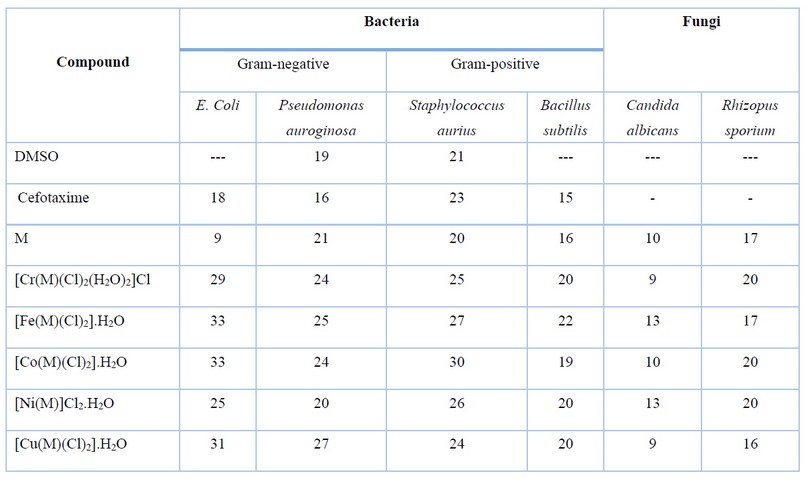
Table 4. The biological evaluation screening data of M and its complexes.
CONCLUSIONS
The synthesis and characterization of a bisaldehyde ligand (M) and its new metal complexes are reported. The tetradentate ligand, which consists of two carbonyl-oxygen and two ether-oxygen donor atoms, was prepared in a two-step. The reaction of the ligand with Cr(III), Fe(II), Co(II), Ni(II) and Cu(II) metal ions in a 1:1 (L:M) mole ratio resulted in the isolation of monomeric complexes. The chemical structure of compounds and the overall bonding behavior of the complexes was confirmed through physicochemical techniques. The characterization data confirmed the isolation of six-coordinate monomeric complexes of the general formula; [Cr(M)(Cl)2(H2O)2]Cl, [M(M)(Cl)2].H2O (where M= Fe(II), Co(II) and Cu(II)) and four-coordinate complex of the general formula [Ni(M)]Cl2.H2O. The biological activity of the ligand and its complexes against bacterial species and fungi pathogens were also tested. Generally, the bisaldehyd complexes showed more activity compared with the free ligand
Author Contributions: Duaa S. Abaas: Performed All Experiments, Data Curation, and manuscript Writing. Mohamad J. Al-Jeboori: Supervision, Methodology, Project Administration, Conceived the Experimental Plan, Analysed the Data, Writing- Original draft, Writing- Reviewing and Editing.
Acknowledgments: The authors are grateful to the University of Baghdad, College of Education for Pure Science (Ibn Al-Haitham) and Department of Chemistry for providing Ms DSA with the MSc studentship and labs facilities.
Conflicts of Interest: The authors declare there are no conflicts of interest.
REFERENCES
1- Holland, J. P.; Hickin, J. A.; Grenville-Mathers, E.; Nguyen, T.; Peach, J. M. Rapid decomplexation of bis (thiosemicarbazonato) zinc (ll) complexes using citric acid. Journal of Chemical Research, 2008, 12, 702-703.
2- Genta, M. T.; Villa, C.; Mariani, E.; Loupy, A.; Petit, A.; Rizzetto, R.; Morini, F.; Ferro, M. Microwave-assisted preparation of cyclic ketals from a cineole ketone as potential cosmetic ingredients: solvent-free synthesis, odour evaluation, in vitro cytotoxicity and antimicrobial assays. International Journal of Pharmaceutics, 2002, 231(1), 11-20.
3- Mounika, K.; Pragathi, A.; Gyanakumari, C. Synthesis characterization and biological activity of a Schiff base derived from 3-ethoxy salicylaldehyde and 2-amino benzoic acid and its transition metal complexes. Journal of Scientific Research, 2010, 2(3), 513-523.
4- Freeman, A.; Woodley, J. M.; Lilly, M. D. In situ product removal as a tool for bioprocessing. Bio/technology, 1993, 11(9), 1007-1012.
5- Ali, S. M. H.; Yan, Y. K.; Lee, P. P.; Khong, K. Z. X.; Sk, M. A.; Lim, K. H.; Klejevskaja, B.; Vilar, R. Copper (II) complexes of substituted salicylaldehyde dibenzylsemicarbazones: synthesis, cytotoxicity and interaction with quadruplex DNA. Dalton Transactions, 2014, 43(3), 1449-1459.
6- Hasan, S.; Salleh, S.; Hamdan, S.; Yamin, B. Unsaturated 15 and 16 Membered Appended Naphthalene Macrocyclic Molecules for The Development of Fluorometric Chemosensors. Conference Series: Materials Science and Engineering, 2017, 172, 1-11.
7- Hussain, S. A.; Al-Jeboori, M. J. New metal complexes derived from Mannich-base ligand; Synthesis, spectral characterisation and biological activity. Journal of Global Pharma Technology, 2019, 11(2), 548–560.
8- Al-Qazzaz, A. H.; and Al-Jeboori, M. J. New metal complexes derived from Mannich ligands; synthesis, spectral investigation and biological. Biochemical and Cellular Archives, 2020, 02(20), 4207-4216.
9- Akkurt, M.; Mohamed, S. K.; Horton, P. N.; Abdel-Raheem, E. M.; Albayati, M. R. 2, 2′-[Ethane-1,2-diylbis(oxy)]dibenzaldehyde. Acta Crystallographica Section E, 2013, 69(8), o1260-o1260.
10- Ali, A.; Merza, J. Synthesis and Characterization of Novel Dialdehydes based on SN2 Reaction of Aromatic Aldehyde. Inorg Chem Ind J.,2017, 2(1), 111-119.
11- Ilhan, S.; Temel, H. Synthesis and spectral studies of macrocyclic Cu (II), Ni (II) and Co (II) complexes by template reaction of 1,4-bis (3-aminopropoxy) butane with metal (II) nitrate and salicylaldehyde derivatives. Journal of Molecular Structure, 2008, 891(1-3), 157-166.
12- Nair, M. S.; Arish, D.; Johnson, J. Synthesis, characterization and biological studies on some metal complexes with Schiff base ligand containing pyrazolone moiety. Journal of Saudi Chemical Society, 2016, 20, S591-S598.
13- Al-Jeboori, M. J.; Abdul-Ghani, A. J.; Al-Karawi, A. J. Synthesis and structural studies of new Mannich base ligands and their metal complexes. Transition Metal Chemistry, 2008, 33(7), 925-930.
14- Zayed, E. M.; Zayed, M. A.; Fahim, A. M.; El‐Samahy, F. A. Synthesis of novel macrocyclic Schiff’s‐base and its complexes having N2O2 group of donor atoms. Characterization and anticancer screening are studied. Applied Organometallic Chemistry, 2017, 31(9), 1-7.
15- Abdul-Ghani, A. J.; Al-Jeboori, M. J.; Al-Karawi, A. J. Synthesis and characterisation of new N2S2 and N2O2 Mannich base ligands derived from phosphinic acid and their metal complexes. Journal of Coordination Chemistry, 2009, 62(6), 2736-2744.
16- Al-Rubaye, B. K.; Brink, A.; Miller, G. J.; Potgieter, H.; Al-Jeboori, M. J. Crystal structure of (E)-4-benzylidene-6-phenyl-1,2,3,4,7,8,9,10-octahydrophenanthridine. Acta Crystallographica Section E: Crystallographic Communications, 2017, 73(7), 1092-1096.
17- Muat, T, H.; Al-Jeboori, M. J. Phenoxo- and azido-bridged complexes with N2OS2 Schiff-base ligand; synthesis, spectral investigation and bacterial activity, ChemXpress. 2016, 9(2), 156-171.
18- Yousif, E. I.; Ahmed, R. M.; Hasan, A. H.; Al-Fahdawi, A. S.; Al-Jeboori, M. J. Metal Complexes of Heterocyclic Hydrazone Schiff-bases; Preparation, Spectral Characterisation and Biological Study, Iranian Journal for Science and Technology, Trans. A, 2017, 41, 103–109.
19- Ye, Y.; Jiang, Z.; Xu, Z., Zhang.; X., Wang, D.; Lv, L.; Pan, B. Efficient removal of Cr (III)-organic complexes from water using UV/Fe (III) system: negligible Cr (VI) accumulation and mechanism. Water Research, 2017, 126, 172-178.
20- Lever, A. Electronic spectra of dn ions. Inorganic Electronic Spectroscopy. 2nd Edt., Elsevier, Amsterdam, 1984; pp. 376-611.
21- Munde, A. S.; Jagdale, A. N.; Jadhav, S. M.; Chondhekar, T. K. Synthesis, characterization and thermal study of some transition metal complexes of an asymmetrical tetradentate Schiff base ligand. Journal of the Serbian Chemical Society, 2010, 75(3), 349-359.
22- Al-Shaalan, N. H. Synthesis, characterization and biological activities of Cu (II), Co (II), Mn (II), Fe (II), and UO2 (VI) complexes with a new Schiff base hydrazone: O-Hydroxyacetophenone-7-chloro-4-quinoline hydrazone. Molecules, 2011, 16(10), 8629-8645.
23- Al-Jeboori, M. J.; Al-Jebouri,. F. A.; Al-Azzawi, M. A. Metal complexes of a new class of polydentate Mannich bases; synthesis and spectroscopic Characterisation, Inorganica Chimica Acta, 2011, 379, 163–170.
24- Ahmed, R. M.; Yousif, E. I.; Al-Jeboori, M. J. Co(II) and Cd(II) complexes derived from heterocyclic Schiff-bases: synthesis, structural characterisation, and biological activity. The Scientific World Journal, 2013, 2013, 1-6.
Received: 2 January 2023/ Accepted: 19 April 2023 / Published:15 June 2023
Citation: Abaas, D. S.; Al-Jeboori, M. J. Coordination Compounds of Carbonyl Oxygen Polychelate Ligand; Synthesis, Physicochemical Characterisation and Biological Evaluation. Revis Bionatura 2023;8 (2) 18. http://dx.doi.org/10.21931/RB/2023.08.02.18