Vol 8 No 2 2023 – 1
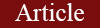
Development of a novel microdevice for sorptive extraction under a green analytical chemistry approach: Application for bioanalytical determination of Ibuprofen

1 Departamento de Control Químico, Facultad de Ciencias Químicas y Farmacia, Universidad Nacional Autónoma de Honduras, Ciudad Universitaria, Tegucigalpa, Honduras; jesica.riera@unah.edu.hn . ORCID
* Correspondence: henry.ponce@unah.edu.hn ,Tel.: +504 32950273
Available from: http://dx.doi.org/10.21931/RB/2023.08.02.1
ABSTRACT
This study proposes a novel device called micro polymeric magnetized bar adsorptive extraction (µ-PMBAE), as an extraction and enrichment technique for trace analysis in aqueous samples. These micro-bar absorbent devices were prepared using easily accessible and low-cost materials, incorporating different sorbent materials into a polymer and introducing a small piece of neodymium magnet. Several stability tests were applied to determine their robustness, stability, and weight variation; finally, ibuprofen concentration in urine samples was determined, demonstrating the applicability of extraction devices under a green analytical chemistry approach. The µ-PMBAE showed remarkable advantages since different sorbents can be added to the hot melt glue. This tunes the interaction with the analytes to become more specific in the extraction. Likewise, the magnetic ability makes it possible to carry out extraction without using an extra stir bar, matching its performance with the traditional commercially based on polydimethylsiloxane phase. The proposed analytical method demonstrated excellent performance concerning high recovery percentages, adequate coefficients of variation, and low detection limits.
Keywords: Bioanalytics, sample preparation, HPLC, µ-PMBAE, green analytical chemistry
INTRODUCTION
In the last three decades, sample treatment techniques have shown an advance in the green chemistry point of view, searching for new methodologies friendly to the environment, with less waste, toxic solvent volumes, energy consumption and timeless development1. The current approach for sample treatment techniques aims to increase the analysis’s selectivity and sensitivity, extract, purify, concentrate and/or derivatize the analytes without using laborious and tedious procedures, with a high economic and time cost such a case of conventional extraction techniques2.
An influential group of microextraction techniques is those that use the mechanism of sorption of the analytes in a solid, which may include absorption (also known as a partition) and adsorption, within which Solid-phase microextraction (SPME) and Stir bar sorptive extraction (SBSE) is found. SBSE, introduced by Pat Sandra in 1999, and marketed under the name of «Twister®» (GERSTEL-GmbH & Co. KG), emerged under the idea of overcoming some of the disadvantages of SPME, including the recovery of analytes with ranges of medium-high volatility present in liquid phase samples3. Likewise, SBSE improves the limited recovery that can be achieved in ultra-trace analysis with SPME, especially in unfavorable phase relationships when working with small volumes of sorptive material, usually, polydimethylsiloxane (PDMS), which covers molten silica fiber4.
In SBSE, stirring bars coated with PDMS is used, with a length of 10 and 40 mm covered with 55 and 219 µL of PDMS, respectively. The stir bars are placed in contact with the liquid sample with stirring times until the analytes are extracted5. Subsequently, in the desorption stage, the analytes are released from the bar, either by a thermal desorption mechanism mainly for gas chromatography or liquid desorption for liquid chromatography. In both stages, variables such as solution volume, speed and stirring time, pH, ionic strength, type, and amount of desorption solvent should be optimized6.
Nowadays, the application of the SBSE has had fundamental advances in using different coating materials, which has achieved the application of the technique for analytes of different polarities7. Gorji et al. developed a monolithic auto-magnetic nanocomposite (SMNM) kit, which proved helpful for determining Bisphenol A in liquid samples8. Other authors have evaluated polydimethylsiloxane-phenyl siloxane as a coating material for extracting antibiotic compounds in aqueous samples9. Along the same lines, W. Fan and collaborators demonstrated the use of coating the bars with an ionic liquid incorporated through the sol-gel route to study naproxen, ketoprofen, and fenbufen in water samples10.
Despite the problems involved in the study of biological samples, where the analytes are at low levels of concentration with the presence of many impurities, which can influence the determination of analytes, the use of SBSE has demonstrated its application in this field. In that sense, using a monolithic acrylate polymer was satisfactory for determining angiotensin II antagonist receptors in human plasma11. Likewise, determining fluoxetine in plasma using a homemade bar by Marques et al. 12. Other works have determined several compounds in urine samples, including fluorouracil and phenobarbital13 and amphetamines14.
Due to the great popularity of the technique, new devices have been developed following the principle of the SBSE. In 2009 J.M.F. Nogueira and his group introduced the use of an analytical device called bar adsorptive µ-extraction (BAµE), for which hollow cylindrical tubes of polyethylene (15 mm in length and 3 mm in diameter) are coated with adhesive films. Then solids Sorbents are adhered to by manual agitation15,16. Likewise, Liu and collaborators developed a device covering a coal pencil mine with a layer of poly luminol by electrochemical polymerization. This obtained good results in extracting residues of β-agonists in pork17. Recently, a tube of polyether ether-ketone treated with sulfuric acid and on whose surface polydopamine and p-naphthol benzene molecules were immobilized was used to determine dye contaminants in environmental samples18.
On the other hand, eight bisphenol compounds were determined by applying a novel cage shell device manufactured in 3D printing consisting of two pieces locked together by a magnetic stirring rod. The authors use nanofibers as adsorbent material19. A new extraction mode that combines the principles of SBSE and DSPE, called stir bar sorptive-dispersive microextraction (SBSDE), was proposed by Benede et al. In the procedure, magnetic nanoparticles were physically loaded on the magnetic stir bar to extract UV absorbent in seawater samples20. In recent years, new trends in SBSE include advances in developing new adsorbent materials, structural changes, and developments in technical automation21.
In the present work, we have developed a device called «micro polymeric magnetized bar adsorptive extraction (µ-PMBAE)» 22, using easy and accessible materials such as hot melt glue, commonly called «termocola», which is composed of polymeric mixtures, with the characteristic of melting at temperatures above 80 °C. It returns to its solid state at room temperature. This property allows mixing the hot melt glue with sorbents usually used for the extraction of analytes, and subsequently, said mixture can be molded of desired size and shape to obtain the adsorptive microbars. Finally, during the elaboration of the device, a neodymium magnet is incorporated into it.
To demonstrate the applicability of the developed devices, it was proposed to determine Ibuprofen as a representative molecule in urine samples. Different sorption parameters were optimized, including stirring time, solution pH, volume, and ionic strength. In the liquid desorption, the sonication time, elution solvent, and volume were evaluated to obtain the most excellent recovery of the analyte. As far as the authors know, this work is the first to develop SBSE devices with the proposed materials, which demonstrate advantages in terms of cost, applicability, easy preparation, and versatility.
MATERIALS AND METHODS
Reagents and Materials
Acetonitrile and methanol HPLC grade, ethyl acetate and hexane pesticide grade, sodium chloride, and sodium hydroxide all of them were obtained from JT BAKER (Center Valley, PA) instead of formic acid, glacial acetic acid, hydrochloric acid, activated charcoal and silica from Merck (Darmstadt, Germany). C18, strong cation exchange (SCX) and weak exchange cationic resin (WCX) sorbents of Agilent (Santa Clara, CA). Ibuprofen standard from Sigma-Aldrich (St. Louis, MO) was used. Ultrasonic baths from Branson (Sterling Heights, MI), vortex mixer from Kool Lab Kyrios-Soter Scientific (Miami, FL), and centrifuge from LW Scientific (Lawrenceville, GA) were used. Stir plate and ultra-pure water obtained with Barnstead micro-pure st from Thermo Scientific (Waltham, MA). For pH measurement, a pH meter from Hanna Instruments HI 9829 (Woonsocket, RI) and a convection oven from Quincy Lab (Chicago, IL) were used for heating in the preparation of the microbars. Hot melt glue sticks and wooden molds were obtained at a local store.
Experimental settings
Preparation of polymeric bars
For the preparation of the devices, the following procedure was performed. An amount of hot melt glue, consisting mainly of ethylene-vinyl acetate copolymer, was melted in a water bath. Subsequently, the sorbent and a few milliliters (≈ 2 mL) of methanol were added to ensure a homogeneous mixture. Then the mixture was subjected to manual stirring, and once the methanol evaporated, the mixture was removed and placed in a wooden mold. It is placed in the oven at 100 °C for 5 minutes. Subsequently, the neodymium magnet is incorporated into the molds and compacted to obtain the desired cylindrical shape. Finally, the molds are removed from the oven, and once they are cooled to room temperature, the formed bars are obtained, which are cut to the desired size (Figure 1. A).
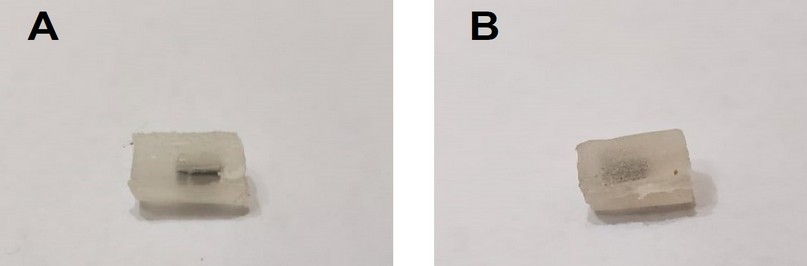
Figure 1. Photography of the µ-PMBAE device (A) without sorbent; (B) with sorbent
Stability and robustness studies
Once the polymeric microbars were prepared, their robustness, stability, and weight variation were evaluated, for which tests were carried out with different solvents, pH values, temperature, sonication process, and sorbent losses as a function of time. Devices prepared with other sorbents were immersed in HPLC vials containing solvents of broad polarity, including hexane, ethyl acetate, acetonitrile, methanol, and aqueous solutions of formic acid and acetic acid, both at 1 %. The microbars were left in the solvents for 48 hours at room conditions. Likewise, to evaluate the effect of pH on the devices, these were immersed in solutions of 1N hydrochloric acid (pH ≈ 2) and 1 N sodium hydroxide (pH ≈10) and left standing at ambient conditions for 48 hours.
The stability depending on the temperature and sonication process was carried out by placing the micro bars in HPLC vials with ultra-pure water and subjecting sonication with varying times of 30, 60, 90, and 120 minutes, measuring the temperature at each of these times. Finally, the devices were weighed immediately after elaboration for the weight variation test, and the average value, standard deviation, and coefficient of variation were obtained. After thirty days, they were again weighed, and different statistical tests were applied to assess their suitability.
Extraction procedure
Once the parameters of the adsorption and desorption processes, including the agitation time, modifier, ionic strength, type and solvent amount of desorption, and sonication time, were optimized until the optimal recovery conditions, the extraction of urine samples was carried out as follows. 2 mL of urine sample was diluted to 20 mL with ultrapure water in a 25 mL glass flask, then the µ-PMBAE was added. The solution was acidified with a 0.1 % (v/v) formic acid solution, and 600 µL of a 25 % (w/v) sodium chloride solution was added. Then the flask was placed under stirring for 2 hours at room condition. After this time, the device was removed, avoiding contamination, and placed in an HPLC vial, and 1 mL of methanol was added and placed in an ultrasonic bath for 60 minutes. Finally, µ-PMBAE is removed, and an amount of the solution is chromatographed by duplicate. Figure 2 shows the optimized microextraction procedure using the µ-PMBE developed in this work.
Chromatographic conditions
The chromatographic separation was carried out on a Shimadzu Prominence liquid chromatograph, consisting of an LC20-AT pump, CMB-20Alite control system, SIL-20A automatic injector, CTO-A20 column oven, and SPD-M20A detector. The EZ Start software was used to collect all the data obtained. A Thermo scientific Hypersill, C8 column (150 x 4.6 mm, 5 µm) was used. In isocratic mode, the mobile phase was a mixture of acidified water with formic acid (pH = 3) and acetonitrile (70:30). The flow of the mobile phase was 1.5 mL/min and injection volume of 5 µL for standard solutions and samples. The DAD was controlled at 220nm. The analyte identification was performed through retention times and comparing the absorption spectrum obtained from the standard.
RESULTS
Preparation of µ-PMBAE devices
The initial objective was to design and build stir bar extraction devices that were made with simple, cheap, and easily accessible materials and that functionally show similarities and, if possible, some advantages with the «Twister» stir bar device, as well as devices recently reported in the literature. The first issue to be defined as the support to which to incorporate the sorbent solid, which could adhere to the sorbent by itself and an excellent malleability to take the desired cylindrical shape and size. The hot melt glue, commonly referred to as «termocola or silicone glue,» is used to adhere various materials; it is easily accessible and has been widely used, and has the characteristic of melting at temperatures above 80 °C, returning to a solid state at room temperature. This glue contains mixtures of polymers, mainly the elastomeric copolymer ethylene vinyl acetate (CAS: 24937-78-81), which has also been used as a drug carrier for 3D printed medical devices of indomethacin due his properties of melting index and flexural23.
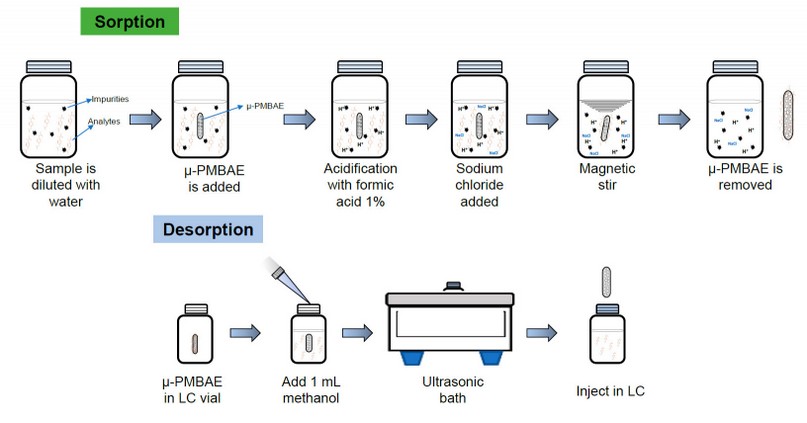
Figure 2. Optimized microextraction procedure using the µ-PMBE to determine Ibuprofen in urine samples.
These characteristics make it an ideal support to incorporate solids on its surface. In the initial tests, modified silica (C18) was integrated, evaluating different percentages of the sorbent, from equal parts of sorbent and hot melt glue to the ratio of 1:0.2. The visual evaluation of the devices obtained showed little homogeneity of the mixture of equal amounts and better with the 1: 0.2 ratio, however, to incorporate a more significant amount of sorbent, which will allow increasing the extraction of the analytes, the amount maximum sorbent with adequate homogeneity was reached when eight parts of the sorbent were mixed in 10 of the glue (ratio 1: 0.8).
To facilitate the homogeneity of the mixture, it was placed at 80 °C under mechanical agitation; here, adding a few milliliters of methanol improved the uniformity. Under these conditions, the organic solvent was evaporated during the heating process. Once this mixture was obtained, giving the devices the appropriate size and shape was necessary. For this, wooden molds with dimensions equal to the size required for the devices were used. Due to the malleability of the polymer, at this stage, an attempt was made to incorporate a magnetic piece so that the use of Teflon-coated magnetic bars could be dispensed with, necessary in other devices reported in the literature, offering this way a similar performance to «Twister stir bar.» For this, cylindrical-shaped nickel-plated neodymium magnets (diameter 2 mm, length 4 mm) were purchased through an e-commerce platform.
When this process was standardized, it was repeated with other sorbents of a wide range of polarity, including activated charcoal, silica, strong cation exchange (SCX), and weak cation exchange (WCX) resins, which are typically used for extraction process due to his sorptive properties. Figure 1.B shows a photograph of the µ-PMBAE device with C18 sorbent; as can be seen, there is a uniformity in the composition of the mixture of the hot melt glue with the sorbent, which could qualitatively guarantee the repeatability of the sorption process. Figure 3 shows a diagram of the µ-PMBAE device.
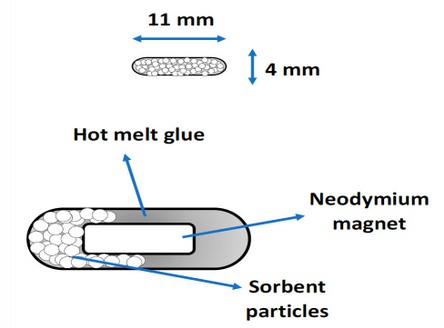
Figure 3. Diagram of the µ-PMBAE device.
Stability tests for µ-PMBAE devices
The next step was to evaluate the stability and robustness of devices developed through appropriate physic-chemical tests based on procedures applied by other authors who report the development of similar devices. This should be done before the application of µ-PMBAE to extract real samples. In the first instance, the µ-PMBAE devices were submitted to the effect of several solvents with a wide range of polarity characteristics, including hexane, acetonitrile, methanol, aqueous solutions of formic and acetic acid at 1 % v/v, regarding the interaction and mainly the occurrence of possible desegregation phenomena of the mixture of hot melt glue and the sorbents. The prepared sorptive bars were immersed for 48 hours in the solvents evaluated under ambient conditions. Then, after this time, a visual examination of the devices was performed. Complete stability in all solvents except for hexane was shown. This can be explained due to the solubility of the copolymer ethylene vinyl acetate in non-polar solvents like hexane.
For the evaluation of the pH effect in the µ-PMBAE devices, these were immersed for two days in solutions of hydrochloric acid (pH ˃ 2) and sodium hydroxide (pH < 10); values outside this range were discarded since they are not usually used to modify the pH of the matrices subjected to extraction. All µ-PMBAE bars showed excellent stability without loss of sorbent particles. The effects of stirring and sonication processes on the stability of the devices were evaluated. For the stirring process, all the prepared µ-PMBAE devices were submitted for 24 hours, showing adequate strength without loss of the sorbents. Likewise, the devices were subjected to an ultrasonic bath for 30 to 120 minutes for sonification tests. A remarkable stability behavior was obtained for all devices. This is especially important for their future application in real samples since both parameters must be optimized to get a high recovery value.
Finally, the variation in the mass of the devices prepared with C18 sorbent was evaluated. For this, a batch of 21 units was prepared and immediately weighed. After 30 days, the mass values were weighted again. The t-test for equal variances showed that there were no significant differences between the initial data with those obtained one month later (tcal = 0.15 < ttab = 1.68), therefore stability in the composition of the µ-PMBAE was demonstrated, without losses of the sorbent as a function of time. From the results obtained in the weight variation test, under the preparation conditions of the devices, it is possible to estimate the amount of sorbent present in each microbar, being more significant than 80 milligrams. (Supplementary Materials).
Application of µ-PBAE in urine samples
Since its inception, SBSE has been widely applied for analyzing biological samples in clinical investigations, bioanalysis, and forensic toxicology. Here, the complexity of the matrix represents a significant challenge to overcome, as well as lower concentration levels of analytes and the small amount of sample available24. Therefore, once the µ-PMBAE had been produced and its stability had been demonstrated, it was decided to use it for the extraction of Ibuprofen, a nonsteroidal anti-inflammatory drug (NSAID) widely used for the treatment of inflammation and pain, as a representative molecule in real urine samples. For chromatographic separation, the conditions established in some previous works in the literature were taken as a basis25,26.
Optimization of the extraction process
In general, for all sample treatment techniques, especially those based on the sorption principle, optimization of his parameters to obtain a high extraction efficiency is required. So, the extraction conditions for the sorption and desorption processes were optimized, starting with the sorbent phase in µ-PMBAE. For this, initial conditions with aqueous solutions of Ibuprofen with a concentration equal to 5 µg / mL (10 mL) were tested and subjected to extraction with the microbars prepared with the five study sorbents. The extraction assemblies were stirred for 6 hours. Subsequently, the devices were removed and desorbed with 1 mL of acetonitrile in an ultrasonic bath for 30 minutes. After this time, the solution was injected. As shown in Figure 4, a higher recovery percentage was obtained with the µ-PMBAE prepared with C18 sorbent, which can be explained due to the low polarity of Ibuprofen (log Kow = 3.97). Under the evaluated conditions, the rest of the sorbents showed recoveries below 25%, so the following tests were performed with the C18 µ-PMBAE.
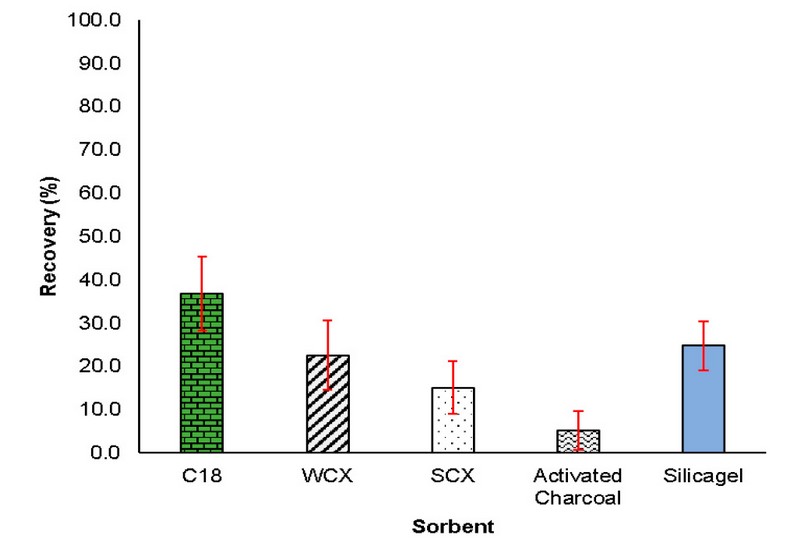
Figure 4. Recovery yields were obtained with the sorbents studied under initial conditions (n=2).
According to Nogueira16, the parameters to optimize in SBSE include solution volume, stirring time and speed, salting effect, pH, and addition of organic modifier for the sorption stage. On the other hand, for liquid desorption, solvent type, volume, and sonication time can be optimized. Table 1 shows the parameters and variables optimized in the present study.
The volume of the solution turned out to be an essential parameter, obtaining better results when 20 mL of total volume was used, which can be explained by the greater contact of the analyte with the µ-PMBAE. Since Ibuprofen has a pKa value equal to 5.3, the pH of the extraction solution plays a vital role in its dissociation, so better results were obtained when acidification with a formic acid aqueous solution was performed; in comparison, when the extraction solution had a pH equal to 7. Although Ibuprofen does not show as a completely hydrophilic compound, modification of ionic strength by adding a few microliters of sodium chloride solution favored the salting-out phenomenon, so a lightly better recovery percentage was obtained. Finally, no significant differences were shown for stirring time between 2 and 6 hours, which means that the equilibrium is reached passed that time. In this line, the stirring speed was not tested since the used stirring apparatus only works at a speed of 1000 rpm.
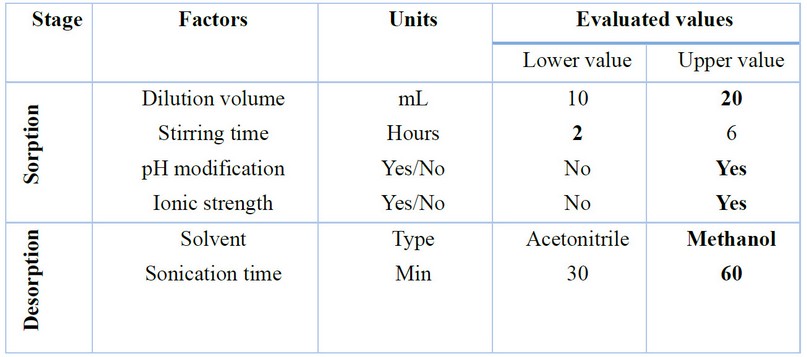
Table 1. Factors and values were evaluated to optimize the extraction efficiency using a 2 6-1 factorial design. Bold values represent the optimized values.
In the liquid desorption stage, a better recovery percentage was obtained using methanol instead of acetonitrile; also, as expected, a better result was observed with 60 minutes of sonication. Other conditions used as a strategy to lower detection limits, such as sample volume and desorption volume, were evaluated. For this, a urine sample taken from a healthy adult volunteer free of ibuprofen intake in the last month was filtered and fortified at three concentrations, 25, 5, and 2.5 µg / mL. Two millimeters of the samples were extracted under optimized conditions and finally desorbed with 1 mL of methanol; the calculated recovery was 96.0 ± 6.5 for high concentration, 87.2 ± 5.4 for the intermediate value, and 79.0 ± 7.7 for the lower (n = 3). Under these conditions, the detection limit was set at 0.5 µg / mL, which can be reduced if the desorption is carried with 0.5 mL of methanol. Figure 5 shows representative chromatograms obtained during the optimization of the method. The specificity of the process was demonstrated by comparing the corresponding UV absorption spectrums for standard solutions and samples (Figure 5).
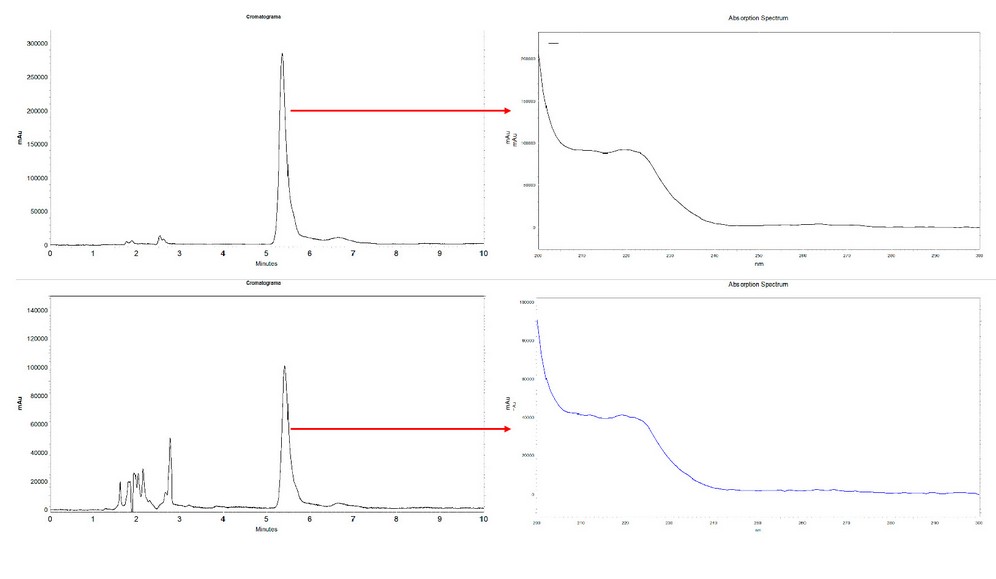
Figure 5. Representative chromatograms. Upper: analysis of standard solution at 50 µg / mL. Lower: analysis of a fortified sample at 25 µg / mL. Images on the right side show the corresponding UV absorption spectrum.
Reuse of the µ-PMBAE devices
At this point, the possibility of reusing µ-PMBAE was considered. For this, using devices to extract standard solutions was left immersed in methanol for 24 hours and subsequently analyzed, finding the presence of Ibuprofen in a range of 2 – 7 % of the fortified amount. Therefore, the reuse of the devices is not recommended unless they are subjected to thorough cleaning. In any case, the low-cost manufacturing of the µ-PMBAE counteracts its non-reuse. Finally, the interaction of the analyte with micro bars without the sorbent phase, composed solely of the polymer, was studied by repeating the extraction of standard solutions with a concentration of 25 µg / mL under optimal conditions. The results showed recoveries close to 25 %, so it is possible to establish that there is an interaction of the analyte with the polymer of the hot melt, possibly under an adsorption mechanism in the polymeric walls, which represents an advantage in its use.
Analytical performance
A fast evaluation of the main analytical performance parameters of the developed method was carried out, including linearity, limits of detection and quantification, recovery, precision, specificity, and selectivity. Adequate linearity was shown in the 50 to 2.5 µg / mL concentration range. The limit of detection (LOD) was calculated based on a signal-to-noise (S/N) ratio greater than 3 and the limit of quantification S/N of 10. The obtained values were 0.5 and 1.7 µg / mL, respectively. As mentioned in the previous section, the recovery yield was obtained at three levels of concentration, established from the peak area of the analyte in the urine sample spiked and the calibration equation obtained in the linearity evaluation. The recovery values obtained were between 79 and 96%. Specificity and selectivity were established by analyzing blank and spiked urine samples, which showed the nonappearance of endogenous substances interferences at the Ibuprofen retention time. Furthermore, the absorption spectrum of the peak attributed to Ibuprofen in the sample was compared with that obtained from the standard solution.
Analysis of actual urine sample
A urine sample from a healthy adult volunteer who was administered a 600 mg dose of Ibuprofen orally was taken three hours after ingestion. The sample was filtered and analyzed in triplicate under the optimized conditions. The concentration found was 23.25 ± 0.51 µg / mL. These results were similar to the findings previously reported27.
µ-PMBAE performance versus other SBSE devices
µ-PMBAE devices developed in this work demonstrate higher performance under optimized experimental conditions for the extraction of Ibuprofen in real urine samples, and its comparison with other devices, including the traditional stir bar, shows some notable advantages concerning economic cost, preparation, and versatility, as shown in Table 2. It is important to note that incorporating the neodymium magnet in the microbars offers the possibility of dispensing with Teflon-coated magnetic bars as in other works reported in the literature, equating its performance to the traditional stir bar device.
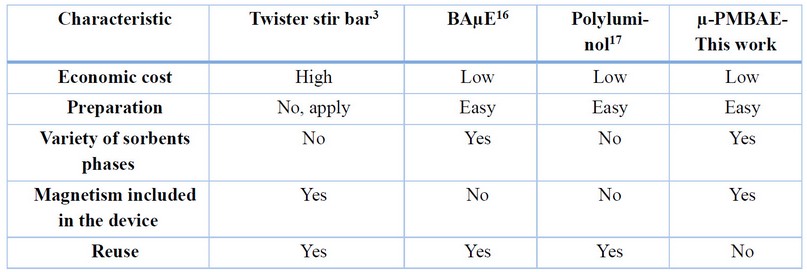
Table 2. Comparison of µ-PMBAE versus other SBSE devices.
DISCUSSION
Applying microextraction techniques based on the green analytical chemistry approach represents an essential trend for developing bioanalytical methods. In this study, a microdevice able to use different sorbents, which are added to a polymer, was developed, which makes it possible to increase the selectivity of extraction. Some of the advantages of this device compared to other devices reported in the literature include a simple manufacturing process, being able to make up to thirty units in less than 30 minutes; low cost per unit (less than one US dollar); and mainly the ability to add different sorbent materials, which translates into more excellent selectivity in the analysis. On the other hand, it is appropriate to point out that the device cannot be reused since a percentage of the extracted analyte remains in the microbar, as discussed in the results section. However, due to its low cost and considering that the neodymium piece can be recovered and reused to manufacture new devices, the authors consider that it does not represent a significant disadvantage. It should be noted that to ensure the composition of the polymer used in the manufacture of µ-PMBAE, it is recommended to prepare the polymer in the laboratory and not use commercial products. This final consideration should be assessed in the future. The analytical methodology was satisfactory for determining Ibuprofen in urine samples, demonstrating an analytical performance with adequate specificity, linearity, precision, and accuracy. Similarly, µ-PMBAE can be used to determine other bioanalytical molecules for which various sorbents, including metallic and vegetable nanoparticles, are promising. For example, our research group uses black carbon nanoparticles from olive seeds to determine dexamethasone in biological matrices.
CONCLUSIONS
The present work reports the development of a new device for sorptive extraction. Using easily accessible and low-cost materials, we developed a novel micro-bar absorbent device, incorporating different sorbent materials into a polymer commonly used as hot melt glue and introducing a small piece of neodymium magnet. The µ-PMBAE device demonstrated excellent stability, robustness, and high extraction efficiency to apply in the preparation of biological samples, following the principles of green analytical chemistry. The developed device has significant advantages compared with other devices reported in the literature. Different sorbents can be stuck on the hot melt glue, which tunes the interaction with the analytes to become more specific in the extraction. Besides, including a piece of a neodymium magnet in the device makes it possible to carry out extraction without needing an extra stir bar, matching its performance with the traditional commercially based on polydimethylsiloxane phase.
Patents
Invention patent Application No.HN/P/2022/001998.
Supplementary Materials: The following are available online at www.noem1.sg-host.com/xxx/s1, Table S1: Summarized results of stability studies., Table S2: Individual weight of µ-PBAE devices.
Author Contributions: Conceptualization, Jessica P. Riera-Williams, and H.D. Ponce-Rodríguez; methodology, Jessica P. Riera-Williams and H.D. Ponce-Rodríguez; software, Jessica P. Riera-Williams and H.D. Ponce-Rodríguez; validation, Jessica P. Riera-Williams and H.D. Ponce-Rodríguez; formal analysis, Jessica P. Riera-Williams and H.D. Ponce-Rodríguez; investigation, Jessica P. Riera-Williams and H.D. Ponce-Rodríguez; resources, H.D. Ponce-Rodríguez data curation, H.D. Ponce-Rodríguez; writing—original draft preparation, Jessica P. Riera-Williams and H.D. Ponce-Rodríguez; writing—review and editing, H.D. Ponce-Rodríguez; visualization, Jessica P. Riera-Williams and H.D. Ponce-Rodríguez; supervision, H.D. Ponce-Rodríguez; project administration, H.D. Ponce-Rodríguez; funding acquisition, H.D. Ponce-Rodríguez. All authors have read and agreed to the published version of the manuscript.
Funding: This research received no external funding
Institutional Review Board Statement: Not applicable.
Conflicts of Interest: The authors declare no conflict of interest.
REFERENCES
1. Gałuszka, A.; Migaszewski, Z.; Namieśnik, J. The 12 principles of green analytical chemistry and the SIGNIFICANCE mnemonic of green analytical practices. TrAC, Trends Anal. Chem., 2013, 50, 78-84. https://doi.org/10.1016/j.trac.2022.116530
2. David, F.; Ochiai, N.; Sandra, P. Two decades of stir bar sorptive extraction: A retrospective and future outlook. TrAC, Trends Anal. Chem., 2019, 112, 102-111. https://doi.org/10.1016/j.trac.2018.12.006
3. Baltussen, E.; Sandra, P.; David, F.; Cramers, C. Stir bar sorptive extraction (SBSE), a novel technique for aqueous sam-ples: Theory and principles. J. Microcolumn Sep., 1999, 11, 737-747. https://doi.org/10.1002/(SICI)1520-667X(1999)11:10<737::AID-MCS7>3.0.CO;2-4
4. Liu, Y.; Liu, Z.; Xu, Z.; Li, g. Stir Bar Sorptive Extraction Technology. Progress in Chemistry, 2020, 32, 1334-1343. http://doi.org/10.7536/PC200101.
5. Mohamed, H.M. Solventless Microextration Techniques for Pharmaceutical Analysis: The Greener Solution. Front. Chem. 2022, 9,785830. http://doi.org/10.3389/fchem.2021.785830.
6. Kabir, A.; Locatelli, M.; Ulusoy, H.I. Recent Trends in Microextraction Techniques Employed in Analytical and Bioanalytical Sample Preparation. Separations, 2017, 4, 36. http://doi.org/10.3390/separations4040036.
7. He, M.; Wang, Y.; Zhang, Q.; Zang, L.; Chen, B.; Hu, B. Stir bar sorptive extraction and its application. J. Chromatogr. A, 2021, 1637, 461810. https://doi.org/10.1016/j.chroma.2020.461810.
8. Gorji, S.; Bahram, M.; Biparva, P. Optimized Stir Bar Sorptive Extraction Based on Self-Magnetic Nanocomposite Monolithic Kit for Determining Bisphenol A in Bottled Mineral Water and Bottled Milk Samples. Anal. Bioanal. Chem. Res., 2019, 6, 137-156. https://doi.org/10.22036/ABCR.2018.143530.1235.
9. Pérez-Padilla, Y.; Medina Cetina, S.A.; Ávila-Ortega, A.; Barrón-Zambrano, J.A.; Vilchis-Néstor, A.R.; Carrera-Figueiras, C.; Muñoz-Rodríguez, D. J. Evaluation of Polydimethylsiloxane-Phenylsiloxane as a Coating for Stir Bar Sorptive Extraction. J. Mex. Chem. Soc., 2018, 62, 348-357. https://doi.org/10.29356/jmcs.v62i2.431
10. Fan, W.; Mao, X.; He, M.; Chen, B.; Hu, B. Development of novel sol–gel coatings by chemically bonded ionic liquids for stir bar sorptive extraction—application for the determination of NSAIDS in real samples. Anal. Bioanal. Chem., 2014, 406, 7261-7273. https://doi.org/10.1007/s00216-014-8141-9
11. Babarahimi, V.; Talebpour, Z.; Haghighi, F.; Adib, N.; Vahidi, H. Validated determination of losartan and valsartan in human plasma by stir bar sorptive extraction based on acrylate monolithic polymer, liquid chromatographic analysis and experimental design methodology. J. Pharm. Biomed. Anal., 2018, 153,204-213. https://doi.org/10.1016/j.jpba.2018.02.030.
12. Marques, L.A.; Nakahara, T.T.; Madeira, T.B.; Almeida, M.B.; Monteiro, A.M.; de Almeida Silva, M.; Carrilho, E.; Piccoli de Melo, L.G.; Nixdorf, S.L. Optimization and validation of an SBSE–HPLC–FD method using laboratory‐made stir bars for fluoxetine determination in human plasma. Biomed. Chromatogr., 2019, 33, 4398. https://doi.org/10.1002/bmc.4398.
13. Ghani, M.; Ghoreishi, S.M.; Shahin, M.; Azamati, M. Zeolitic imidazole framework templated synthesis of nanoporous carbon as a coating for stir bar sorptive extraction of fluorouracil and phenobarbital in human body fluids. Microchem. J., 2019, 146, 798-806. https://doi.org/10.1016/j.microc.2019.02.007
14. Taghvimi, A.; Dastmalchi, S.; Javadzadeh, Y. Novel Ceramic Carbon-Coated Magnetic Nanoparticles as Stir Bar Sorptive Extraction Coating for Simultaneous Extraction of Amphetamines from Urine Samples. Arab J Sci Eng., 2019, 44, 6373-6380. https://doi.org/10.1007/s13369-019-03810-0
15. Neng, NR; Silva, A.R.M.; Nogueira, J.M.F. Adsorptive micro-extraction techniques—Novel analytical tools for trace levels of polar solutes in aqueous media. J. Chromatog. A, 2010, 1217, 7303-7310. https://doi.org/10.1016/j.chroma.2010.09.048
16. Ahmad, S.M.; Nogueira, J.M.F. High throughput bar adsorptive microextraction: A novel cost-effective tool for monitoring benzodiazepines in large number of biological samples. Talanta, 2019, 199, 195–202. https://doi.org/10.1016/j.talanta.2019.02.004
17. Liu, H.; Ousmane, D.; Gan, N.; Wu, D. Li. Novel Stir Bar Array Sorptive Extraction Coupled with Gas Chromatography–Mass Spectrometry for Simultaneous Determination of Three β2-Agonist Residues in Pork. Chromatographia, 2017, 80, 473-482. https://doi.org/10.1007/s10337-017-3242-1
18. Zhou, W.; Wang, C.; Wang, X.; Chen, Z. Etched poly(ether ether ketone) jacket stir bar with detachable dumbbell-shaped structure for stir bar sorptive extraction. J. Chromatogr. A, 2018, 1553, 43-50. http://doi.org/10.1016/j.chroma.2018.04.022.
19. Šrámková, I.H.; Horstkotte, B.; Erben, J.; Chvojka, J.; Švec, F.; Solich, P.; Šatínský, D. 3D-Printed Magnetic Stirring Cages for Semidispersive Extraction of Bisphenols from Water Using Polymer Micro- and Nanofibers. Anal. Chem. 2020, 92, 3964–3971. https://doi.org/10.1021/acs.analchem.9b05455.
20. Benedé, J.L.; Chisvert, A.; Giokas, D.L.; Salvador, A. Development of stir bar sorptive-dispersive microextraction mediated by magnetic nanoparticles and its analytical application to the determination of hydrophobic organic compounds in aqueous media. J. Chromatogr. A, 2014, 1362, 25–33. https://doi.org/10.1016/j.chroma.2014.08.024.
21. Hasan, C.K.; Ghiasvand, A.;Lewis, T.W.; Nesterenko, PN;Paull, B. Recent advances in stir-bar sorptive extraction: Coatings, technical improvements, and applications. Anal. Chim. Acta, 2020, 1139, 222-240. https://doi.org/10.1016/j.aca.2020.08.021
22. Invention patent Application No.HN/P/2022/001998.
23. Genina, N.; Holländer, J.; Jukarainen, H.; Mäkilä, E.; Salonen, J.; Sandler, N. Ethylene vinyl acetate (EVA) as a new drug carrier for 3D printed medical drug delivery devices. Eur. J. Pharm. Sci., 2016, 90, 53-63. https://doi.org/10.1016/j.ejps.2015.11.005
24. Madej, K.; Piekoszewski, W. Modern Approaches to Preparation of Body Fluids for Determination of Bioactive Compounds. Separations, 2019, 6, 53. https://doi.org/10.3390/separations6040053
25. Ascar, L.; Ahumada, I.; López, A.; Quintanilla, F.; Leiva, K. Nonsteroidal Anti-Inflammatory Drug Determination in Water Samples by HPLC-DAD under Isocratic Conditions. J. Braz. Chem. Soc., 2013, 24, 1160-1166. http://doi.org/10.5935/0103-5053.20130150.
26. Ramos Payán, M.; Bello López, MA; Fernández Torres, R.; Villar Navarro, M.; Callejón Mochón, M. Electromembrane extraction (EME) and HPLC determination of nonsteroidal anti-inflammatory drugs (NSAIDs) in wastewater samples. Talanta, 2011, 85, 394-399. http://doi.org/10.1016/j.talanta.2011.03.076.
27. Jain, B.; Jain, R.; Kabir, A.; Sharma, S. Rapid Determination of Nonsteroidal Anti-Inflammatory Drugs in Urine Samples after In-Matrix Derivatization and Fabric Phase Sorptive Extraction-Gas Chromatography-Mass Spectrometry Analysis. Molecules, 2022, 27, 7188. https://doi.org/10.3390/molecules27217188.
Received: 10 February 2023/ Accepted: May 15 2023 / Published:15 June 2023
Citation: Riera-Williams J P, Ponce-Rodríguez H D. Development of a novel microdevice for sorptive extraction under a green analytical chemistry approach: Application for bioanalytical determination of Ibuprofe. Revis Bionatura 2023;8 (2) 1. http://dx.doi.org/10.21931/RB/2023.08.02.1