Vol 8 No 1 2023 – 12
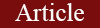
Characterization of the microbial community associated with the roots of joyapa (Macleania rupestris) and the effect of fungal isolates on seedling development
Diana Curillo 1*, Juan Manuel Cevallos-Cevallos 2 , Eduardo Chica 1 and Denisse Peña 1
1 Facultad de Ciencias Agropecuarias, Universidad de Cuenca, 010201, Cuenca, Ecuador.
2 Centro de Investigaciones Biotecnológicas del Ecuador (CIBE), Escuela Superior Politécnica del Litoral, ESPOL, 090505, Guayaquil, Ecuador.
* Correspondence: diana.curillos@ucuenca.edu.ec
Available from: http://dx.doi.org/10.21931/RB/2023.08.01.12
ABSTRACT
Macleania rupestris is a native Ericaceae with high potential benefits for health and the environment. Characterizing the interactions between M. rupestris and associated fungi is vital to supporting the plant’s conservation and future domestication. However, little is known about the relationship between plant growth-promoting endophytes and M. rupestris. To learn more about the soil-associated microbiota of M. rupestris, we analyzed endophyte communities associated with the plant’s rhizosphere and surrounding soil using nanopore sequencing. Additionally, fungal endophyte cultivable strains were isolated from the roots of M. rupestris to evaluate their growth-promoting activity on seedlings by applying a strain inoculation bioassay. Over 1000 genera were identified using nanopore sequencing, Bradyrhizobium and Mesorhizobium the most abundant in all samples analyzed.
Similarly, six cultivable fungi were characterized by the molecular markers ITS (internal transcribed spacer) and LSU (large subunit). Amongst all isolates, Clonostachys rosea and Trichoderma paravidescens positively impacted seedlings’ development. This study shows the potential of fungal strains as inoculants for the potential domestication of Macleania rupestris.
Keywords: Endophyte, growth promoter, Macleania rupestris, microbial communities
INTRODUCTION
Ericaceae is considered one of the most prominent families of angiosperm, plants that include herbs, dwarf shrubs, shrubs, and trees commonly found in acidic and infertile growing conditions 1. In the Andean region of Ecuador, there is an excellent variety of Ericaceae used by rural and indigenous communities. Among them, Macleania rupestris, known locally as «joyapa» is a species of Ericaceae that provides fruits for human consumption in communities of the Andean region2,3. The Ericaceae family is one of the most representatives of the Ecuadorian Andean forests, mainly in the montane cloud forests, as well as in the paramo and sub-paramo regions4, where these plants play critical ecological roles such as a water regulator, a food source for several birds species and other animals such as the Andean bear; and, in some cases, a source of income for the inhabitants of the region 2. Additionally, M. rupestris have the potential to be used in recovery projects for degraded areas 5. Even though the importance of M. rupestris to its habitat, very little is known about the conservation and propagation of this species.
Mycorrhizal endophytic associations are complex fungal-derived traits that help develop plant physiology6,7. In other Ericaceae species, various endophytes have been examined for their ability to produce growth-promoting metabolites like those made by their hosts, but in more significant quantities 8,9. However, the information regarding these symbiotic associations in M. rupestris is minimal 10, so a complete characterization of these fungal communities is required to know more about the conservation of M. rupestris.
This study contributes to the knowledge of the complex associations established by M. rupestris in the Andes of southern Ecuador. We set out to characterize the endophytic communities of rhizospheric soil and soil surrounding M. rupestris seedlings in specimens collected in three locations in the southern highlands of Ecuador province Azuay. To better understand the effect of endophyte fungi, we isolated and identified cultivable fungal strains from M. rupestris roots to later evaluate their effect on the initial development of M. rupestris seedlings germinated under laboratory conditions.
MATERIALS AND METHODS
Location and sampling
Three sampling points were established within the province of Azuay, Ecuador, in the parishes of Baños (2°57’25.1″S 79°05’46.8″W), Sayausí (2°51’12.5″S 79°06′ 10.4″W) and Sigsig (3°00’51.7″S 78°46’48.0″W), located at altitudes between 2900 and 3100 masl. The collected samples were processed in March 2021 at the Faculty of Agricultural Sciences of the University of Cuenca as described below.
Soil sample collection
The soil samples were collected from the roots of M. rupestris, in the seedling stage, using the protocol described by Barrilot et al. 11, which consists of digging an approximate depth of 30 cm to obtain the samples; approximately 200 g of soil was collected. To get surrounding soil samples, part of the roots of the joyapa seedlings selected in the field were extracted and shaken vigorously for 10 min to collect the soil. Additionally, the rhizospheric soil samples were obtained manually by removing the soil that remained attached to the roots of the seedlings. Separately collected soil samples from the surrounding soil were sieved and stored in plastic bags in the dark at approximately 4°C, using ice. All samples were transported to the laboratory and stored at -20 °C until analysis.
DNA extraction from soils and metagenomic sequencing
To characterize the microbial community associated with the surrounding soil and rhizosphere of M. rupestris, Nanopore MinION Oxford sequencing was carried out 12. DNA extraction was performed using 250 mg of soil, previously stored at -20 °C. The extraction was performed using the DNeasy PowerSoil Pro Kit (Qiagen, Hilden, Germany), according to the manufacturer’s instructions. The quality and concentration of the DNA were evaluated using the QubitTM 4.0 fluorometer (Thermo Fisher Scientific, 2018).
Libraries for MinION sequencing were prepared using the 1D Ligation Sequencing Kit (SQK-LSK109), and the DNA from each sample was barcoded using the Native Barcode Expansion Kit (EXP-NBD103) (Oxford Nanopore Technologies, Oxford, UK) 13,14. All kits were used following the manufacturer’s instructions. The libraries were then sequenced in a MinION Mk1B for 6 hours using an R9 flow cell. Data was processed using the Oxford Nanopore Technology (ONT) cloud-based pipeline EPI2ME (WIMP rev. 3.2.3) workflow for WIMP (what is in my pot) analysis to obtain the microbial community information down to the genus level as well as some species.
Statistical analysis
The α diversity of the community was estimated using the indices of Shannon, Simpson and Chao1. Beta diversity was estimated using Bray-Curtis dissimilarity, employing the verdict function of the vegan package in R. Then, permutational multivariate analysis of variance (PERMANOVA) was used to compare the microbial community structure between surrounding and rhizospheric soil; this was done with 999 permutations using the adonis function from the vegan package in R.
Isolation of endophytic fungi
M. rupestris roots previously collected and stored at 4 °C were disinfected by washing with running water, then immersed in a 70 % (v/v) ethanol solution for 1 min, followed by a 1.5% sodium hypochlorite solution for 5 minutes. Finally, the roots were rinsed three times with sterile distilled water 10. Then, a fragment of approximately 3 cm of the disinfected root was placed in a sterile Petri dish and cut into small pieces with the help of tweezers and a scalpel. Sterile FIM culture medium (Fungi Isolation Medium) was added to the fragments obtained. The Petri dishes were then closed and kept at room temperature (around 20 °C) for 96 hours, observing the emergence of hyphae daily and transferring them to PDA medium (potato dextrose agar) for further development; PDA petri dishes were incubated at room temperature for 5 days. Fully grown fungi were classified according to color and appearance 10,15.
DNA extraction from isolated fungal strains
Genomic DNA extraction was performed by adding 500 µl of TE buffer pH 8.0 to 50 mg of mycelium collected from the PDA culture dishes, then centrifuging at 15,000 rpm for 5 min and removing the TE buffer, adding 300 µl of extraction solution (200 mM Tris HCl pH 8.5, 250 mM NaCl, 25 mM EDTA 0.5% SDS). The mycelium was then ground in a TissueLyser II (Qiagen) with metal beads for 3 minutes, and 150 µl of 3 M sodium acetate, pH 5.2, was added. The suspension was then incubated at -80°C for 10 minutes, thawed and centrifuged (Eppendorf Centrifuge 5430 R) at 15,900 x g for 5 min. The supernatant was transferred to another test tube, and an equal amount of isopropanol was added. After 5 min of incubation at room temperature, the supernatant was combined with 300 µl of 70% ethanol and centrifuged at 15,900 x g for 15 min to perform a wash 16. Electrophoresis was performed on a 1.5% agarose gel using ethidium bromide to visualize the extracted DNA. To analyze DNA, 2µl of the sample was read through a spectrophotometer (Epoch Biotek Instruments) to determine DNA quantity and quality.
Identification of isolated fungal strains
From the extracted DNA, a fragment of the ITS region was amplified using the primers ITS1 (5′-TCCGTAGGTGAACCTGCGG-3′) and ITS4 (5′-TCCTCCGCTTA TTGATATGC-3′)17 as well as the large subunit (LSU) region using the primers LR0R (5′-ACCCGCTGAACTTAAGC-3′) and LR3 (5′-CCGTGTTTCAAGACGGG-3′)18. The amplified fragments were sequenced by an external service provider (Macrogen Inc., South Korea) and then compared to the NCBI (National Center for Biotechnology Information) GenBank database using BLAST 19. The identity of each isolate was assigned based on the percentage of similarity with the sequences found in the GenBank database.
Germination of seeds and obtaining seedlings
Ripe fruits of M. rupestris were collected between the months of January – March. Fruit disinfection was carried out in a laminar flow hood, for which the fruits were immersed in a 70% (v/v) ethanol solution for 1 min and then in a sodium hypochlorite solution (3.5%) for 10 min. Finally, the fruits were rinsed three times with sterilized distilled water20. The seeds were then extracted with the help of a syringe needle and placed in a 2000 ppm AG3 (gibberellic acid) solution for 24 h at 20 ºC.
Subsequently, the seeds were placed in a 9-cm diameter Petri dish with filter paper and sterile distilled water for 8 weeks to germinate and obtain seedlings. The seedlings were placed in sterile peat for the inoculation tests using the fungi previously isolated from the roots of M.rupestris.
Inoculation of fungal strains in M. rupestris seedlings
A total of six isolated fungi were selected to evaluate their effect on the initial development of M. rupestris seedlings. After two weeks of adaptation of the seedlings in the sterile peat, the inoculation of the different strains of isolated fungi was carried out, for which a solution prepared with half a Petri dish of mycelium diluted in 250 ml of sterile distilled water was applied21.The seedling growth assay was carried out under controlled conditions in a growth room for 90 days under a photoperiod of 16 hours of light and 8 hours of darkness at 25 ± 2ºC with 10 individual seedlings for each isolated endophytic fungus and the non-inoculated control group. All seedlings were treated with conventional organic fertilizer with nutrient solution and then inoculated with fungi isolates except for the control group. The number of roots and the height of the seedlings were recorded 90 days after inoculations. Plant height was measured from the soil surface to the tip of the stem. Plant growth data were subjected to one-way ANOVA (α = 0.05)22.
RESULTS
Microbial communities present in the surrounding and rhizospheric soil of M. rupestris
From the two samples collected (1 surrounding and one rhizospheric), for each of the 3 sampling points, community compositions indicated the presence of 4 kingdoms, 40 phyla, 83 classes, 175 orders, 366 families, 1090 genera and 3151 species in the rhizospheric soil samples and for the surrounding soil, 4 kingdoms, 36 phyla, 72 classes, 156 orders, 330 families, 894 genera and 2432 species were found. It was observed that a total of 15 phyla belonging to the bacteria kingdom presented a relatively high abundance, greater than 1%, in the rhizospheric and surrounding soil samples. According to the data obtained, the Bacteria kingdom was the most abundant, constituting 96% of the total readings. The Fungi kingdom only included 4%, of which none was categorized as an endophyte.
When analyzing the diversity index (Shannon), dominance index (Simpson) and richness index (Chao1) for the bacterial communities in the surrounding and rhizospheric soil, it’s evident that there is greater diversity in the rhizospheric soil of M. rupestris compared to the surrounding soil samples (Figure 1). It was also found that the structure of the microbial community differed from the type of soil sampled (rhizospheric/surrounding), observing an increase in the distance (Bray – Curtis, p = 0.03) between the rhizospheric and surrounding soil samples. (PERMANOVA Shannon, p = 0.05, PERMANOVA Simpson, p = 0.02, PERMANOVA Chao1, p = 0.01).
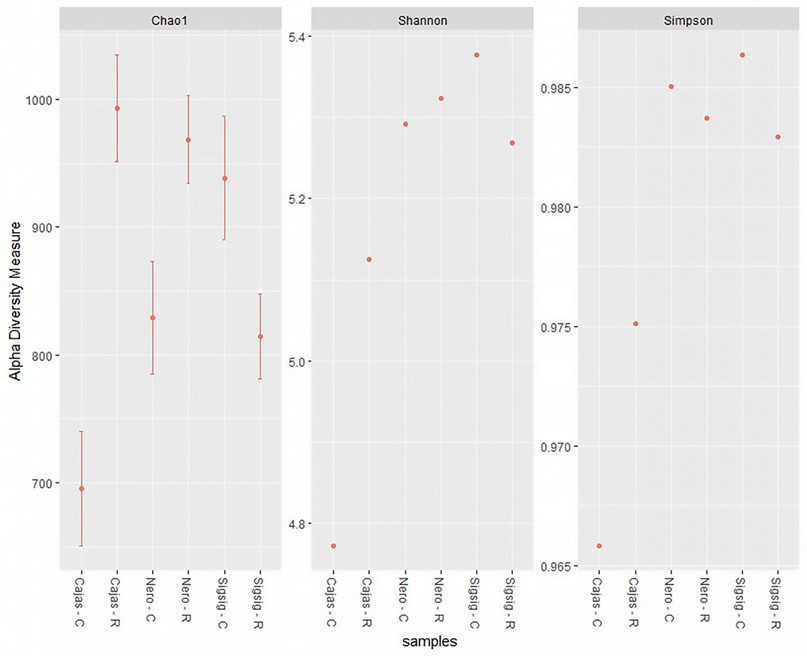
Figure 1. Alpha diversity indices (Chao1, Shannon and Simpson). R = rhizospheric soil C = surrounding soil.
Comparing the rhizospheric soil samples with the surrounding soil samples, the dominant genera (average relative abundance > 1%) in all samples were Bradyrhizobium (16%), Pseudomonas (4.7%), Sphingomonas (3.3%), Mesorhizobium (3 %), Burkholderia (2.8%), Variovorax (2.5%), Paraburkholderia (2.7%),) and Rhodopseudomonas (1.4%). The relative abundance of Bradyrhizobium and Burkholderia in the rhizosphere soil was like the composition of the surrounding soil (Figure 2).
Figure 2. Relative abundance of identified genera for each soil sample. The EPI2ME WIMP workflow analyzed reads. Bacterial genera contributing more than 0.5% of the classified reads are shown. R = rhizospheric soil C = surrounding soil.
Isolation of cultivable fungi
A total of 12 fungal strains were isolated from the roots of M. rupestris. Fungal strains with similar morphotypes were present in most of the cultivated roots, which is why they were grouped according to their morphology (color, shape, and growth time) in a total of six morphotypes, which were used in the subsequent identification and molecular analysis (Figure 3)
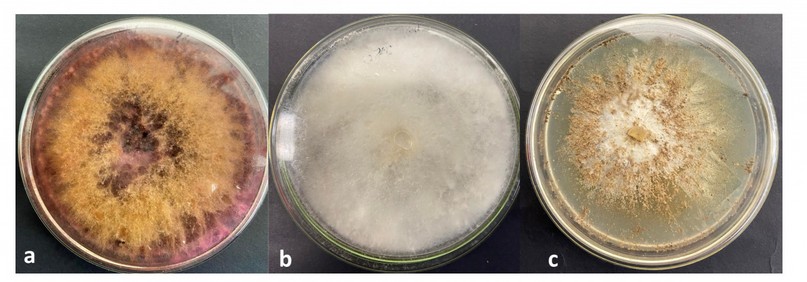
Figure 3. Fungal strains isolated from M. rupestris roots. a.) Fusarium asiaticum b.) Trichoderma paraviridescens c.) Clonostachys rosea
Of the six strains of isolated fungi, 3 belonged to the genus Trichoderma, and included T. paraviridescens, T. viridescens, and T. atroviridae. Additionally, two strains were within the Fusarium genus, and included F. asiaticum and F. graminearum, and finally, a Clonostachys rosea isolate was obtained. All species shared a 99% similarity to those from Genebank (Table 1).
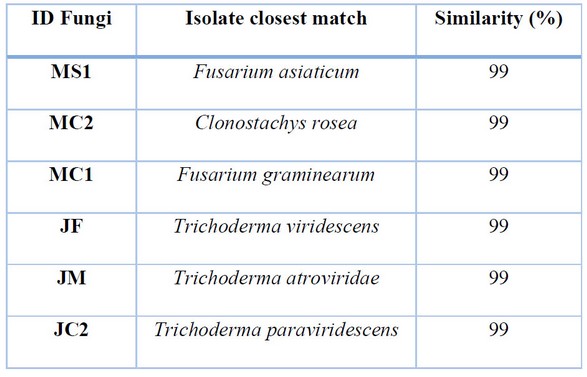
Table 1. Search closest matches of fungal isolates against NCBI Genbank databases.
Inoculation of cultivable endophytic fungi in M. rupestris seedlings
After 90 days, plants inoculated with Clonostachys rosea presented the best results in their development, evaluated by the number of leaves and the height of the seedlings. The seedlings exhibited better development than the control group seedlings (Figure 4a). As for the number of leaves, the average was of 8 per seedling and 6.5 cm in height, unlike the control group, which presented standards of 5 leaves per seedling and 2 cm in height (Figure 4b,c). The results were similar to the seedlings inoculated with T. paraviridescens, which registered 7 leaves and an average size of 5 cm (Figure 4b,c). Except for T. viridescens, all the fungal strains influenced the development of the seedlings, surpassing the control treatment in height, however concerning the number of leaves, in addition to C. Rosea and T. paraviridescens, only T. viridescens, slightly outperformed the control group.
DISCUSSION
Microbial diversity present in rhizospheric and surrounding soils of M. rupestris
Soil microorganisms play a critical role in regulating soil fertility and plant health30. We are beginning to understand how soil microbial diversity varies worldwide and how this diversity is related to the physical, chemical, and biological characteristics of ecosystems31. The study carried out in the surrounding and rhizospheric soil of M. rupestris showed that the diversity of microbial communities could vary significantly concerning the proximity to the root. The metagenomic analysis of the surrounding and rhizosphere soil samples obtained a higher abundance of bacterial communities than fungal communities. This can happen due to several factors, such as changes in the types and amounts of organic carbon in the soil 32,33. Although fungi and other eukaryotes may represent a large part of the soil microbial biomass, their representation in the metagenomic data obtained was low. A similar pattern has been observed in comparable shotgun metagenomic datasets obtained from other soils34,35 and is most likely a product of many eukaryotic taxa (including fungi), which have a much lower ratio of rRNA gene copies per unit biomass than bacterial cells 35.
Analyzing the bacterial communities obtained, we find that bacteria belonging to the genera Bradyrhizobium, Pseudomonas, Sphingomonas, have been previously shown to be the most abundant taxa in the rhizosphere and endosphere of plants 24,36. Therefore, the same taxa are expected to be the most productive in the analyzed samples.
Actinobacteria are a species and class of Gram-positive bacteria and are the group with the highest representation in surrounding soils and the second most represented in rhizospheric soils. This taxon is particularly interesting for its wide biotechnological potential37. Actinobacteria in soils have capacities for mutualism, symbiosis and pathogenesis38.
The most abundant genera within the samples are Bradyrhizobium, Pseudomonas, and Sphingomonas. Bradyrhizobium spp. is an agronomically crucial gram-negative bacterium capable of forming root nodules and fixing atmospheric nitrogen 39. Bradyrhizobium species produce auxins, cytokinins, abscisic acid, vitamins and riboflavin, which stimulate plant growth40. Pseudomonas are important bacteria in agriculture and have been shown to promote growth and protect plants from pathogens41 and are part of the core microbiome of many plants42. These genera present in the soil samples are essential because they are involved in plant growth, which is of utmost importance for the propagation of M. rupestris.
Inoculation of plants with isolated fungi
Endophytic interactions are a complex matrix of symbiotic functions between the host plant and its microbiome. For several years, many endophytic communities and their vital role in the development and growth of Ericaceae have been studied 23,24. In the inoculation test of endophytic fungi in M. rupestris plants, C. rosea presented favorable results in terms of seedling development. C. rosea is reported as an endophytic fungus for various plants such as corn25, wheat26and blueberry27 . It has been reported as a promoter of seed germination and helps plants such as blueberry plants to resist extreme environmental factors like major droughts27. C. rosea has also been reported as a biological controller of Botrytis cinerea in tomato plants 28.
Among the isolated fungi, 3 species of Trichoderma were also found, which also presented favorable results in terms of the number of leaves per plant and plant height; this can be attributed to the fact that fungi of the genus Trichoderma produce glucanases, chitinases and proteases, enzymes that break down the components of the cell wall chitin, polysaccharides and β-glucans, facilitating the obtaining of nutrients for plants28. Trichoderma paraviridescens is also used in crops as growth promoters or for seed germination29.
The results obtained show that the fungi isolated from the roots of M. rupestris did help the leaf development and height of seedlings. C. rosea was one of the fungi with the greatest potential to be used in future studies, with M. rupestris as a growth promoter.
Further research is needed to assess the role of bacteria isolates in seedlings and plants of M. rupestris
.
CONCLUSION
This study is the first to analyze the diversity of microbial communities present in the surrounding and rhizospheric soil of M. rupestris using metagenomic analysis. The data obtained provide information on the microbial distribution in M. rupestris plants and the dominant genera present in the development of the plants, the most prominent groups being Bradyrhizobium, Proteobacteria and Actinobacteria. In conclusion, the rhizospheric soil of M. rupestris has greater microbial diversity than the surrounding soil and a large part of the microbial community is recurrent for these plants, even though they come from different sites, which could indicate a close interrelationship and/or dependence between these plants and some of the microorganisms with which it is associated
The treatments that were inoculated with fungal strains isolated from the roots of plants of the same species showed more significant growth than the control treatment, and even though the effect regarding the number of leaves was not higher in all cases than the control treatment, it can be evidenced the importance of microbial associations in the development of M. Rupestris seedlings, highlighting the effect of C. rosea and T. paraviridescens.
Acknowledge
Thanks to all authors and the Faculty of Agricultural Sciences for all the support to develop this investigation. This research project was carried out within the framework of a joint VLIR NETWORK postgraduate program.
REFERENCES
1 Ștefănescu BE, Szabo K, Mocan A, Crişan G. Phenolic Compounds from Five Ericaceae Species Leaves and Their Related Bioavailability and Health Benefits. Molecules 2019; 24: 2046.
2 Durán-Casas S, Veloza-Suan C, Magnitskiy S, Lancheros HO. Evaluation of uva camarona (Macleania rupestris Kunth A.C. Smith) propagation with air layering. 2013; : 9.
3 Veloza C, Durán S, Magnitskiy S, Lancheros H. Rooting Ability of Stem Cuttings of Macleania rupestris Kunth A.C. Sm., a South American Fruit Species. International Journal of Fruit Science 2014; 14: 343–361.
4 Luteyn JL, Wilbur RL. FLORA COSTARICENSIS Family #172 Ericaceae. 2005; : 105.
5 Ortiz S, Consuegra C, van der Hammen MC, Pérez D. Perspectivas urbano-rurales sobre la circulación de dos frutos silvestres del Bosque Altoandino en sistemas agroalimentarios de Bogotá, Colombia. Revista Etnobiología 2021; 19: 81–95.
6 Hawksworth DL. Fungal diversity and its implications for genetic resource collections. 2004www.indexfungorum.org/names.
7 Wang Z, Li T, Wen X, Liu Y, Han J, Liao Y et al. Fungal Communities in Rhizosphere Soil under Conservation Tillage Shift in Response to Plant Growth. Front Microbiol 2017; 8. doi:10.3389/fmicb.2017.01301.
8 Zhao J, Zhou L, Wang J, Shan T, Zhao J, Zhou L et al. Endophytic fungi for producing bioactive compounds originally from their host plants Spin-wave resonance in (Ga,Mn)As thin films View project Gamma-ray burst polarimeter POLAR View project Endophytic fungi for producing bioactive compounds originally from their host plants. https://www.researchgate.net/publication/229024535.
9 Alurappa R, Chowdappa S, Narayanaswamy R, Sinniah UR, Mohanty SK, Swamy MK. Endophytic Fungi and Bioactive Metabolites Production: An Update. In: Microbial Biotechnology. Springer Singapore: Singapore, 2018, pp 455–482.
10 Hamim A, Miché L, Douaik A, Mrabet R, Ouhammou A, Duponnois R et al. Diversity of fungal assemblages in roots of Ericaceae in two Mediterranean contrasting ecosystems. C R Biol 2017; 340: 226–237.
11 Barillot CDC, Sarde C-O, Bert V, Tarnaud E, Cochet N. A standardized method for the sampling of rhizosphere and rhizoplan soil bacteria associated to a herbaceous root system. Ann Microbiol 2013; 63: 471–476.
12 Leggett RM, Clark MD. A world of opportunities with nanopore sequencing. J Exp Bot 2017; 68: 5419–5429.
13 Huang Z, Liu B, Yin Y, Liang F, Xie D, Han T et al. Impact of biocontrol microbes on soil microbial diversity in ginger ( Zingiber officinale <scp>Roscoe</scp> ). Pest Manag Sci 2021; 77: 5537–5546.
14 Srivastava R, Srivastava AK, Ramteke PW, Gupta VK, Srivastava AK. Metagenome dataset of wheat rhizosphere from Ghazipur region of Eastern Uttar Pradesh. Data Brief 2020; 28: 105094.
15 Declerck S, Séguin S, Dalpé Y. The Monoxenic Culture of Arbuscular Mycorrhizal Fungi as a Tool for Germplasm Collections. In: In Vitro Culture of Mycorrhizas. Springer-Verlag: Berlin/Heidelberg, pp 17–30.
16 Moťková P, Vytřasová J. Comparison of Methods for Isolating Fungal DNA. Czech J Food Sci 2011; 29: 10.
17 Bruns TD, Lee SB, Taylor JW. Amplification and Direct Sequencing of Fungal Ribosomal RNA Genes for Phylogenetics Evolution of Gene Expression View project. https://www.researchgate.net/publication/262687766.
18 Vilgalys R, Hester M. Rapid genetic identification and mapping of enzymatically amplified ribosomal DNA from several Cryptococcus species. J Bacteriol 1990; 172: 4238–4246.
19 Altschul S. Gapped BLAST and PSI-BLAST: a new generation of protein database search programs. Nucleic Acids Res 1997; 25: 3389–3402.
20 TURNER SR, COMMANDER LE, BASKIN JM, BASKIN CC, DIXON KW. Germination behaviour of Astroloma xerophyllum (Ericaceae), a species with woody indehiscent endocarps. Botanical Journal of the Linnean Society 2009; 160: 299–311.
21 Repáč I. Ectomycorrhizal Inoculum and Inoculation Techniques. 2011, pp 43–63.
22 Prasad R. Plant promoting activities of fungal endophytes associated with tomato roots from Central Himalaya, India and their interaction with Piriformospora indica Improvement of water quality of Sitalakhaya River by using hydrodynamic model View project Molecular characterization of bacteria isolated from different environment View project. 2015www.ijpbs.net.
23 Vano I, Sakamoto K, Inubushi K. Selection of dark septate endophytes from Ericaceae plants to enhance blueberry (Vaccinium corymbosum L.) seedling growth. 2010.
24 Yurgel SN, Douglas GM, Dusault A, Percival D, Langille MGI. Dissecting Community Structure in Wild Blueberry Root and Soil Microbiome. Front Microbiol 2018; 9. doi:10.3389/fmicb.2018.01187.
25 Goh YK, Marzuki NF, Tuan Pa TNF, Goh T-K, Kee ZS, Goh YK et al. Biocontrol and Plant-Growth-Promoting Traits of Talaromyces apiculatus and Clonostachys rosea Consortium against Ganoderma Basal Stem Rot Disease of Oil Palm. Microorganisms 2020; 8: 1138.
26 Nagasawa AElise. Evaluation of Clonostachys rosea for growth enhancement and suppression of fusarium seedling blight in wheat. Library and Archives Canada = Bibliothèque et Archives Canada, 2010.
27 Sutton JC, Liu W, Ma J, Brown WG, Stewart JF, Walker GD. EVALUATION OF THE FUNGAL ENDOPHYTE CLONOSTACHYS ROSEA AS AN INOCULANT TO ENHANCE GROWTH, FITNESS AND PRODUCTIVITY OF CROP PLANTS. Acta Hortic 2008; : 279–286.
28 Nordström SA. Endophytic growth of Clonostachys rosea in tomato and Arabidopsis thaliana. http://stud.epsilon.slu.se.
29 Zheng H, Qiao M, Lv Y, Du X, Zhang K-Q, Yu Z. New Species of Trichoderma Isolated as Endophytes and Saprobes from Southwest China. Journal of Fungi 2021; 7: 467.
30 Jacoby R, Peukert M, Succurro A, Koprivova A, Kopriva S. The Role of Soil Microorganisms in Plant Mineral Nutrition—Current Knowledge and Future Directions. Front Plant Sci 2017; 8. doi:10.3389/fpls.2017.01617.
31 Dasgupta D, Brahmaprakash GP. Soil Microbes are Shaped by Soil Physico-chemical Properties: A Brief Review of Existing Literature. Int J Plant Soil Sci 2021; : 59–71.
32 Fierer N, Bradford MA, Jackson RB. TOWARD AN ECOLOGICAL CLASSIFICATION OF SOIL BACTERIA. Ecology 2007; 88: 1354–1364.
33 Goldfarb KC, Karaoz U, Hanson CA, Santee CA, Bradford MA, Treseder KK et al. Differential Growth Responses of Soil Bacterial Taxa to Carbon Substrates of Varying Chemical Recalcitrance. Front Microbiol 2011; 2. doi:10.3389/fmicb.2011.00094.
34 Delmont TO, Prestat E, Keegan KP, Faubladier M, Robe P, Clark IM et al. Structure, fluctuation and magnitude of a natural grassland soil metagenome. ISME J 2012; 6: 1677–1687.
35 Fierer N, Lauber CL, Ramirez KS, Zaneveld J, Bradford MA, Knight R. Comparative metagenomic, phylogenetic and physiological analyses of soil microbial communities across nitrogen gradients. ISME J 2012; 6: 1007–1017.
36 Hacquard S, Garrido-Oter R, González A, Spaepen S, Ackermann G, Lebeis S et al. Microbiota and Host Nutrition across Plant and Animal Kingdoms. Cell Host Microbe 2015; 17: 603–616.
37 Worthen DB. Streptomyces in Nature and Medicine: The Antibiotic Makers. J Hist Med Allied Sci 2007; 63: 273–274.
38 Schrempf H. Actinobacteria within soils: capacities for mutualism, symbiosis and pathogenesis. FEMS Microbiol Lett 2013; 342: 77–78.
39 Li C, Li X, Kong W, Wu Y, Wang J. Effect of monoculture soybean on soil microbial community in the Northeast China. Plant Soil 2010; 330: 423–433.
40 Parihar J, Parihar SP, Suravajhala P, Bagaria A. Spatial Metagenomic Analysis in Understanding the Microbial Diversity of Thar Desert. Biology (Basel) 2022; 11: 461.
41 Adesemoye AO, Kloepper JW. Plant–microbes interactions in enhanced fertilizer-use efficiency. Appl Microbiol Biotechnol 2009; 85: 1–12.
42 Lally RD, Galbally P, Moreira AS, Spink J, Ryan D, Germaine KJ et al. Application of Endophytic Pseudomonas fluorescens and a Bacterial Consortium to Brassica napus Can Increase Plant Height and Biomass under Greenhouse and Field Conditions. Front Plant Sci 2017; 8. doi:10.3389/fpls.2017.02193.
Received: September 26, 2022 / Accepted: October 15, 2022 / Published:15 February 2023
Citation: Curillo D, Cevallos-Cevallos J M , E Chica , Peña D. Characterization of the microbial community associated with the roots of joyapa (Macleania rupestris) and the effect of fungal isolates on seedling development.Revis Bionatura 2023;8 (1) 12. http://dx.doi.org/10.21931/RB/2023.08.01.12