Vol 7 No 4 2022- 66
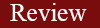
Predominant genetic mutations leading to or predisposing diabetes progress: A Review
Shaima Rabeea Banoon 1*
, Mohammed Younis Alfathi 2
, Seyyed Khalil Shokouhi Mostafavi 3
, Abdolmajid Ghasemian 4**




1 Department of Biology, College of Science, University of Misan, Maysan, Iraq.
2 Department of Biology, College of Education for Pure Science, University of Mosul, Mosul, Iraq.
3- Department of Microbiology, Faculty of Medicine, Tehran Medical Sciences, Islamic Azad University, Tehran, Iran.
4- Noncommunicable diseases Research Center, Fasa University of Medical Sciences, Fasa, Iran.
*Corresponding author: (Shaima Rabeea Banoon *) Email: shimarb@uomisan.edu.iq ; (Abdolmajid Ghasemian ** ) Email: majidghasemian86@gmail.com
Available from: http://dx.doi.org/10.21931/RB/2022.07.04.66
ABSTRACT
Diabetes mellitus (DM) arises following poor capacity to generate or secrete insulin or insulin resistance; hence insulin production impairment creates the illness. Individuals can control their weight, impulsivity, blood pressure, and blood lipids at the commencement of the disease. A single genetic mutation affects nearly 3% of people with diabetes. Surprisingly, beta cell function is regulated by more than 20 genes. Benefits of genetic diagnosis include improved therapy, better prediction of illness prognosis and progression, genetic counseling, and possibly prevention.
Alpha HNF1 mutations in the early stages may respond to the regimen. Still, most patients need it because they control their blood glucose and will be subject to microvascular or macrovascular complications. In cases where insulin does not control sugar, using low-dose sulfonylureas would be beneficial and lower four times the glucose metabolism of metformin. These patients are susceptible to sulfonylureas and may be treated for years in case of no blood glucose attack complications. The drug will start at one-fourth of the adult dose: MODY1. It is caused by a mutation in the alpha-HNF 4 gene and is relatively uncommon. The same is true, but the threshold for renal excretion is not low, and the incidence of upward alpha-HNF 4 mutations in cases where there is a robust clinical panel for alpha HNF 1 but not confirmed by genetic sequencing should be considered. The disease is also susceptible to sulfonylureas: MODY4 with a mutation in the MODY6 gene, IPF1, with a mutation in MODY7, NeuroD1 is characterized by a carboxy sterilise mutation, which is not common: MODY2. In children and adolescents, an increment in fasting blood glucose of 100 to 150 mg/dl is not typical. The incidence of this condition is usually considered to be type 1 or 2 diabetes, but a large percentage of the above patients are heterozygote individuals, the glucokinase mutations. Specific mutations, including those rare variants in WFS1 and ABCC8 genes, insulin receptor (IR), fructose 6-phosphate aminotransferase (GFPT2), and nitric oxide synthase (eNOS), as well as mouse pancreatic β‐cell lines (Min6 and SJ cells), showed that the HDAC4 variant (p. His227Arg) had been directly linked with T2DM.
Keywords: type-2 diabetes, genetic mutations, risk factors
INTRODUCTION
Diabetes is a metabolic disorder that affects the human body 1. The body’s ability to produce or release insulin hormone is lost or becomes insulin-resistant, resulting in decreased insulin production. The primary function of insulin is to reduce blood sugar levels through various processes 2.
Diabetes can be categorized into two types. Pancreatic β-cell degeneration causes defective insulin production in type 1 diabetes. In contrast, in type 2 diabetes, a progressive resistance to insulin occurs in the body that can eventually result in pancreatic -cell degeneration and complete insulin-producing insufficiency. Genetic factors, obesity, and dementia 3 influence type 2 diabetes.
Diabetes impairs the body’s ability to use and metabolize glucose and lower blood glucose levels, culminating in hyperglycemia 2,4. Long-term sugar accumulation in the body destroys very small veins, affecting different organs such as the kidneys, eyes, and nerves 5. Since diabetes raises the risk of cardiovascular disease, screening and early diagnosis of the condition in high-risk people can help prevent these complications 6.
Factors Affecting Type 2 Diabetes
Many factors contribute to the onset of diabetes, some of which can be controlled by individuals, such as weight, impulsivity, blood pressure, and lipids. Other cases, however, appear to be linked to the disease 7. For instance, if someone in your family has diabetes, you are also at risk of developing it (which points to genetic influences in the disease) 7,8. Some surveys have found that certain breeds, including blacks, Spaniards, and American Indians, are more likely to show the impact of race on the disease 9. The risk of developing diabetes, however, rises with age. The prevalence of diabetic patients with the polycystic ovarian syndrome was shown to be higher (PCOS) in females 10-12.
Gestational Diabetes
Any elevation in blood glucose during pregnancy reaching a high level of 5-10% of the population is called gestational diabetes 1. It has been determined that pregnancy itself can be one of the causes of diabetes. This effect is due to increased body resistance to insulin and increased insulin to compensate for this problem. Pregnancy can reveal even mild deficiencies of insulin secretion, leading to glucose intolerance and gestational diabetes13. On the other hand, some patients with mildly diabetic glucose are screened during pregnancy under this group 4. Gestational diabetes involves 3-8% of pregnancies and is also one of the risk factors for poor pregnancy outcomes. This condition can also cause type 2 diabetes. Research has outlined that almost half of these women will develop diabetes over the next 20 to 30 years 14. Increased gestational diabetes has been reported in recent years. Several factors have contributed to this increase. These include the high prevalence of obesity among young patients and improving the survival of female children whose birth weight is at the two ends of the normal birth weight range. In adulthood, these children have impaired insulin function or the ability to secrete insulin that can predispose them to gestational diabetes 15. Gestational diabetes can cause serious complications for the mother and the baby. These complications can be mitigated by proper diagnosis and treatment. Women with high risk for 75G glucose tolerance test (OGTT) are tested at the first post-pregnancy visit, and a re-test is implemented at 24 to 28 weeks of pregnancy. Treatment for gestational diabetes is primarily a diet and physical activity, and insulin therapy is used to control sugar in the absence of response. These women are also tested regularly after pregnancy 16.
Other types of diabetes
Genetic defects of β-cells
Diabetes is a complex and heterogeneous condition characterized by persistent hyperglycemia caused by defects in insulin secretion, insulin resistance, or a combination of the two. It has been estimated that roughly 5% of all types of diabetes are caused by these mutations. Nevertheless, accurate diagnosis is vital in treatment, prognosis, and assessment of the risk in the family 17. The most frequent type is usually linked with an increase in low glucose levels (under 25 years of age) and is acknowledged as the most commonly diagnosed diabetes mellitus in young patients (called MODY, which is the acronym for maturity-onset diabetes of the young). This type is an autosomal recessive disorder which affects six chromosomal locations. The mutations mainly occur (50-70 percent of cases) on chromosome 12 in the hepatic transcription factor, also known as HNF-1 mutation 18.
The second one is associated with the glucokinase mutation on the 7p chromosome, leading to the production of a defective glucokinase molecule (the enzyme catalyzes the formation of glucose-6-phosphate) and stimulation of insulin secretion. Higher glucose levels are required for normal insulin secretion due to this mutation. Other gene transcription factors with sporadic mutations include HNF-4α, HNF-1β, IPF-1 and NeuroD1 19. Genetic tests for this type are commonly used in cases where the incidence of diabetes is low and unusual symptoms associated with type 1 diabetes and 2 are observed or a strong family history of this type is recommended 20. Maternally Inherited Diabetes and Deafness (MIDD) is caused by an alteration or mutation in mitochondrial DNA (the most frequent change is known as 3243A>G) and was first identified in the early 1990s 21. The disease is associated with diabetes and deafness 22. The most common form of mutation in position 3243 is in the tRNA of the leucine gene. A similar lesion is observed in MELAS (Mitochondrial encephalopathy, lactic acidosis, and stroke-like episodes); however, diabetes is not part of the syndrome 23. In small families, genetic disorders resulting in the inability to convert proinsulin to insulin have also been observed. This is inherited as an autosomal dominant trait. In this case, the glucose disorder is mild 24. Neonatal diabetes mellitus is another heterogeneous group of diabetics that occurs up to 6 months of age and has an approximate incidence of 1:100,000 live births with variations within different ethnic groups. Several mutations that interrupt the process of pancreatic organogenesis, the formation of -cells, and the production of insulin cause the disorder. Abnormalities of the 6q24 region and mutations of the genes coding for the ATP-dependent potassium channel are the most common genetic causes of neonatal diabetes with normal pancreatic shape 17.
Disorders of the insulin function
Genetic disorders of insulin function include unusual cases of diabetes. Metabolic disorders caused by these mutations comprise hyperinsulinemia, mild hyperglycemia, and severe diabetes. Some patients with these defects may have nigricans acanthosis 25. Women may exhibit male body traits and have cystic ovaries. In the past, this syndrome was considered an insulin resistance type. Leprechaunism (also known as Donohue syndrome) and Rabson-Mendenhall are two syndromes in children that contain mutations in insulin receptors resulting from severe insulin resistance 26.
Outbreaks of pancreatic bronchitis
Diabetes is one of the chronic complications of chronic pancreatitis. The difference in this type of diabetes is the destruction of the pancreatic endocrine, and therefore the glucagon secretion also mitigates; thus, in these diabetic patients, the risk of hypoglycemia (a decrease in blood sugar) is sought after treatment 27. Apart from pancreatitis, diabetes can be a complication of any damage to the pancreas, including infections, pancreatic surgery, and pancreatic cancer 28.
Endocrine disorders
Several insulin-like hormones participate, and excessive discharge can lead to diabetes initiation. Usually, this situation is observed in patients predisposed to diabetes due to defective diabetes insulin secretion 29. Increased growth hormone and cortisol are commonly caused by hormonal disorders that lead to diabetes, resulting in increased complications and cardiovascular mortality in these diseases owing to diabetes. These hormonal disorders have been attributed. It is estimated that 16-56% of patients have acromegaly, and a 20-50% increase in diabetes mellitus occurred in Cushing’s syndrome 30.
Diabetes through drugs or chemicals
Irreversible degeneration of β-cells may occur in rare cases following the administration of mouse poison vapor or intravenous pentamidine. Some medications can also interfere with insulin function. For example, nicotinic acid and glucocorticoids are from this category. Patients taking interferon-alpha also have antibodies to pancreatic anesthetics or severe insulin deficiency in some cases 31. Some high-performance and relatively safe drugs are associated with an increased risk of diabetes, including anti-hypertension, statins and beta-blockers 32. Regarding statins studies have exhibited that this increase in risk is meager, and at present, this increase in trouble does not justify stopping or reducing statin use 33.
Rare types
Infections: Some infections such as measles, congenital cytomegalovirus, and coxsackie virus subtypes by 3 and 4 34.
Types of Rare immunity: Stiff man’s syndrome, anti-insulin-receptor antibodies 35.
Other genetic syndromes that are associated with increased risk of diabetes include Down syndrome, Klinefelter syndrome, Turner syndrome, Wolfram Syndrome, Friedreich’s ataxia (FRDA), Huntington’s Disease, Laurence-Moon-Bardet-Biedl syndrome (LMBBS), Myotonic Dystrophy, Porphyria, and Prader Willi Syndrome (PWS) 36.
Informal categories
The last official categorization of diabetes was by the American Diabetes Association in 1997, and despite its problems, it is still underlined by the main authorities 37. However, diabetes has long been characterized by a heterogeneous disease and may require a new division. Below are some suggestions for categorization:
Type one and a half diabetes
Latent Autoimmune Diabetes of Adults (LADA), a subtype of type I that has characteristics of type 2 diabetes, is supposed to be one and a half in between the two types of diabetes 38. Those under age 50 are slimmer, have a history of autoimmune diseases, and will likely require insulin within 5 years after the onset of the disease 39.
Type 3 diabetes
Epidemiologic and scientific evidence suggests a common pathophysiology of type 2 diabetes (T2DM) and Alzheimer’s disease (AD). As far as the hypothesis is concerned that AD may be «type 3 diabetes». Researchers have found that insulin resistance being the main marker as a mechanism for diabetes, also occurs in the brain. It is worth considering that, unlike insulin-dependent members, the muscle, the liver, and the fatty tissue, the brain is one of the insulin-independent organs, not requiring insulin to enter glucose into the cells. Then they disclosed that the resistance is associated with Alzheimer’s disease 40. Another study outlined that Alzheimer’s can develop in the brain without hyperglycemia 41.
There is growing interest in detecting the role of insulin genes and insulin-like growth factor (IGF) and its related receptors (T2DM) in developing cognitive impairment, Alzheimer’s disease and Aβ lesions in the brain. This relationship suggests that insulin and the mechanism of insulin signaling are essential for the survival of neurons. Noticeably, studies have exhibited reduced brain growth and increased tau phosphorylation in insulin-receptor-2-impaired mice. On the other hand, various studies have revealed that extractable amyloid beta (ADDL) ligands may also be responsible for this phenomenon. ADDL has similar morphology and size oligomers to prions associated with neurodegenerative diseases. ADDLs may lower insulin levels and insulin resistance in the brain of Alzheimer’s patients. It is concluded that the term «type 3 diabetes» indicates that Alzheimer’s disease is a type of diabetes that selectively involves the brain and has molecular and biochemical properties that overlap with type 1 and type 2 diabetes 41-43.
Signs and symptoms
In the early stages, diabetes may be asymptomatic. Many patients are accidentally diagnosed in a test or during screening. As blood glucose levels enhance, symptoms of diabetes will become more apparent. Hyper-uremia, overdrinking, overeating, and weight loss, despite high appetite, fatigue, and blurred vision, are common symptoms of diabetes. Many patients have had diabetes for several years at diagnosis and even have diabetes complications. In children with type 1 diabetes, symptoms usually appear suddenly; these patients are generally healthy, while not obese previously. In adults, these symptoms tend to be more pronounced. Ketoacidosis can be observed as a symptom of the onset of the disease in type 1 diabetes 44. In type 2 diabetes, an individual is usually asymptomatic for several years. Symptoms are generally mild and gradually deteriorate. Eventually, the person suffers from excessive fatigue and blurred vision and may develop dehydration. In these patients, the incidence of ketoacidosis is lower due to insulin production. However, blood glucose can increase to very high levels, and the person may develop hyper-muscular shock. The results of a study exhibit that stress and depression increase the likelihood of stroke and death from cardiovascular disease in patients with diabetes more than twice 45.
Risk factors for type 2 diabetes
Some risk factors are as follows:
· Age over 40 years
· First-degree family with type 2 diabetes
· A history of pre-diabetic glucose disorders (impaired glucose tolerance, impaired fasting glucose)
· The history of gestational diabetes
· The history of the birth of a baby with macrosomia (overweight)
· The presence of complications of diabetes on the organs of the body
· There are risk factors for cardiovascular disease (such as high blood fat, high blood pressure, and obesity)
· The presence of diabetes-related diseases (polycystic ovarian syndrome, acanthosis nigricans, HIV infection, and some psychiatric disorders such as schizophrenia, depression and bipolar disorder)
· Use of diabetes medications: Corticosteroids, atypical antipsychotics, HIV / AIDS treatment, 46-49.
Pre-diabetic disorders
In patients with type 2 diabetes, diabetes develops gradually over several years. Patients undergo an asymptomatic preparatory phase before reaching clear and distinct diabetes. The group prescribes pre-diabetes as some source of «increased risk of diabetes.» The World Health Organization also calls them «hyperglycemia»50,51.
In this group of patients, the criteria for diagnosis of diabetes, such as fasting plasma glucose FPG or hemoglobin A1C, or oral glucose tolerance test (OGTT), is higher than the normal values and does not go well enough to diagnose diabetes mellitus. Accordingly, in each of the three methods of diagnosis of diabetes, we are confronted with a group that has normal values to diabetic levels in the middle 52.
Regarding fasting blood glucose, the normal value is below 100 mg / dL, and the limit for diagnosis of diabetes is 126 mg / dL. Therefore, patients with fasting blood sugar of between 100 and 125 mg / dL, having dysfunction of pre-diabetics, are called «Impaired fasting glucose» (IFG).
In hemoglobin A1C, normal values are below 5.6%, and the diagnostic limit of diabetes is more significant than 6.5%. Hence, individuals with values between 5.7% and 4.6% are included in the pre-diabetic group.
In the glucose tolerance test, normal blood glucose levels are less than 140 mg / dL two hours after eating 75 milligrams of sugar, whereas, for diabetes, this value should be greater than or equal to 200 mg / dL, which is why this subgroup of patients, whose values range from 140 to 199 milligrams per deciliter, is called «impaired glucose tolerance» (IGT) 53.
Patients in these three groups do not necessarily overlap. In other words, someone may have normal fasting blood glucose but with a diabetic pre-diabetic glucose tolerance test.
The next point is that, in the clinical practice field, the use of a sugar tolerance test, although accurate, is not usually recommended for diagnosis of diabetes, nor pre-diabetic diagnosis, and is most often used for research and epidemiological research. The current criteria are fasting blood glucose or hemoglobin A1C, considered the most appropriate test for asymptomatic individuals 50.
But the most crucial issue in diabetic patients is their outcome and how to deal with this disorder. Patients with type 2 diabetes should have a pre-diabetic condition before they develop diabetes, which will put them at risk of being able to prevent diabetes by appropriate treatment. Today, there is a controversy about the drug treatment of these patients. The American Diabetes Association ADA says that if a predisposed person has high-risk criteria (body mass index BMI greater than 35, a family history of diabetes in first-degree relatives or a woman who has a history of gestational diabetes), it is better to use metformin to prevent the onset of diabetes. However, most patients do not have these criteria and must change their lifestyles. These patients must have a curriculum to lose weight and increase physical activity by regular exercise at least five times a week. These patients should also be tested annually for diabetes, while they, besides diabetes, are at a higher risk for cardiovascular disease and therefore need to be screened for cardiovascular disease 54.
Exercise and diabetes
Exercise helps people with diabetes avoid heart attacks, blindness, and nerve damage. When you eat, your blood sugar levels increase. The more blood levels increase, the more sugar the cells stick. When sugar is attached to the cell, it can no longer be separated and become a harmful substance called sorbitol, which can cause blindness, deafness, brain damage, and burning legs syndrome 55
When sugar is delivered to your body, it can only be stored in the liver and muscle cells. The sugars have no place to go if the liver and muscle cells are saturated with carbohydrates. If the storage of muscle cells is consumed after exercise, after the meal, sugars are absorbed by the muscle, and their amount does not increase in the blood 56.
However, transcription factors seem not to be the only factors involved in differentiating α to β cells. In a study in this regard, stem cells were used to create insulin-producing β cells. However, observations suggest that these cells cannot respond to glucose extracellular stimuli. By inserting these cells into mice’s bodies and maturation of these cells for five months, these cells find the ability to balance the blood sugar relative to the extracellular stimulus of glucose. Epigenetic changes play a significant role in determining the fate of endocrine cells and their full maturity. In other words, transcription factors and epigenetic modifications both play a role in deciding the fate of a cell and a specific feature of that cell 57.
DNA methylation is one of the epigenetic changes contributing to a cell’s survival. Recent studies have exhibited that the role of methylation in α pancreatic cells is not limited to cell survival. In these cells, DNA methylation maintains the specific character of α cells and differentiates them from other endocrine cells. Dnmt1 is one of the enzymes involved in DNA methylation, and the researchers study simultaneously the enzyme and the Arx transcription factor to differentiate α to β cells 58.
Removing the Arx transcription factor alone causes 30% of α cells to become β-like cells after 12 weeks. However, newly created cells do not have specific markers of β-cell maturation. In contrast, with the removal of Dnmt1, none of the particular early factors of β cells occurred after 10 months. In the next step, the removal of both Arx and Dnmt1 factors took place. In the 12th week, the elimination of these two factors resulted in more than 50% of α-cells able to produce insulin, and the expression of specific factors associated with β-maturity was observed. Notably, the newly produced β cells are similar to those of the β core in terms of their physiological properties. As a result of this research, the importance of two factors, Arx and Dnmt1, was identified in maintaining the characteristics of α cells. It was also exhibited that the elimination of these two factors contributes to the specific properties of β cells 59. Diabetes treatment can also be considered. Treatment for diabetic wounds is implemented in different ways:
Foot care: This includes moisturizing the wound environment by choosing the right ingredient, as well as keeping the edges of the wound dry. In patients with insulin-dependent diabetes mellitus, care lasts for about 3 years.
Antibiotics: Antibiotics are prescribed even when infections have not occurred (prophylaxis) to prevent infection.
Blood Glucose Control: One of the causes of diabetic wounds is high blood sugar. High blood glucose reduces immunity and delay wound healing. Blood glucose control, either as a medicine or as a diet, as well as short-term insulin administration, would improve ulcer healing.
Skin transplant: This can also treat diabetic wounds.
Surgery: Removing dead tissue around the wound site is usually implemented to cleanse and heal the wounds. Bypass surgery improves blood flow to the legs, which may help heal the wound and prevent amputation, and it is needed at the end of the amputation to stop the infection from spreading.
Hyperthermia Oxygen Therapy: Increasing the concentration of O2 from 20% to 100% by 5 times and increasing its pressure from 1 atm to 2 atm total leads to a 10-fold increase in oxygen content. One of its effects is the formation of more blood vessels in the area mitigating blood flow and more proper flow to areas that have blocked blood. Hyperbaric oxygen therapy seems to help lower amputation.
American researchers believe that the current treatment of diabetes can help adults to live well with the aging process 60.
Types of Monogenic Diabetes and Their Care
Diabetes mellitus under the age of six months and neonatal diabetes
There is usually no diabetes mellitus in this age range. The HLA analysis also identifies more protective types against type one 61. Neonatal diabetes begins at the beginning of the first three months of life, and insulin needs to control the sugar. Clinically, there are two subgroups for the neonatal subgroup. The transient type of neonatal diabetes is characterized by an improvement of about 12 weeks, though more than 50% of them eventually relapse. This type needs lifelong treatment after diagnosis. There is a neonatal transitional diabetes mellitus and a neonatal mutation gene (ABCC8, KCNJ11 receptor sulfonylurea) 62. For both cases, the use of sulfonylureas may be beneficial. If both parents have glucose intolerance, heterozygote or homozygote mutations are common. At the onset of neonatal diabetes, it can be challenging to detect transient or persistent diabetes. The common cause of transitional neonatal diabetes is a disorder of the gene 6 q24 63. Parental or dystonic polysaccharides and methylation are common disorders. Diabetes mellitus begins in the first week and recovers at 12 weeks. In 50% of cases, diabetes recurs in a child 64.
Macroglossia is observed in 23% of cases. Blood glucose is between 200 and 1000 mg/ dL and is needed to control insulin requirements, but insulin needs to be reduced quickly. In the phase of patient improvement, care should be taken 65. Treatment with sulfonylureas and metformin has not been appropriately evaluated. The second most persistent and transient diabetes infection in the first 6 months of the mutation is Kir 6.2 gene. While 10% may be temporary (although the likelihood of relapse is high), most cases of diabetes are due to this permanent gene deficiency. Most patients only have diabetes, but there may be neurological manifestations in 20% of cases. In 90% of cases, the disease is due to a new mutation. Severe cases may be associated with severe developmental delay and epilepsy, similar to West syndrome. This condition is known as the DEND syndrome (Delay, Epilepsy Neonatal Diabetes Developmental), with a complete clinical profile of insulin dependence, and 30% of them are referred to by ketoacidosis. Peptide C levels cannot be measured, and in these patients with sulfonylureas, The whole face is not treated. Still, relatively good control can be given to them without the risk of hypoglycemia 66. A dose of 0.5 mg/kg is used to manage glibenclamide properly.
Wolcott-Rallison syndrome is characterized by diabetes, episodial dysplasia, renal dysfunction, acute liver failure, and developmental delay with recessive inheritance. This disease is associated with the mutation of E1F2AK3. Diabetes usually precedes early life. (Although there may be delayed onset), and decreasing beta cells and reducing insulin secretion by an immune mechanism 67.
There is a need for insulin for treatment. This diagnosis should be considered in diabetic patients starting at age 3 and with episodial dysplasia or acute renal failure 68.
Familial types of diabetes
MODY3 The possibility of monogenic diabetes in cases where one of the parents is diabetic (type 1 or 2) should be considered 69. The most common form of familial diabetes mellitus is due to the mutated nuclear factor of hepatocytes, alpha-HNF 1, which are characterized by:
▪ Diabetes mellitus that begins in the lower ages but is not insulin-dependent (not associated with ketoacidosis, the low metabolic rate of insulin is produced appropriately). There is a secretion of the C Peptide outside the honeymoon period 70.
▪ There is a family history of diabetes. Diabetes begins in parents at 20-30 or even at 40 and may be treated with either insulin. They may even have one of the grandparents or grandparents 71.
▪ Glucose tolerance testing in the early stages of high blood sugar (usually more than 90 mg). Some patients may have normal fasting blood glucose. Still, at the second glucose test, they reach the diagnostic range of diabetes 72.
Blood glucose is often observed even though blood glucose is average because the threshold for renal excretion is low in patients.
Severe hypersensitivity to sulfonylureas causes hypoglycemia (despite poor glycemic control before the onset of the drug) 73.
Major mutations in diabetes
Mutations are genetic sequence variations that can have a wide range of effects on a person’s health 86. A single genetic mutation affects about 3% of diabetic patients. Surprisingly, more than 20 genes are involved in beta cell activity. Improved therapy, better prediction of disease prognosis and progression, genetic counseling, and possibly prevention are all benefits of genetic diagnosis 74.
Alpha HNF1 mutations in the early stages may respond to the regimen. Still, most patients need it because control of their blood glucose will be confused and subject to microvascular or macrovascular complications. In cases where insulin does not control sugar, low-dose sulfonylureas will be beneficial and lower 4 times the glucose metabolism of metformin 75 (Table 1). These patients are susceptible to sulfonylureas and may be treated for years if they have no problems with blood glucose attacks. Glycemic control with sulfonylureas will be better than insulin to avoid hypoglycemic episodes. The drug will start at one-fourth of the adult dose: MODY1 76. It is caused by a mutation in the alpha-HNF 4 gene and is relatively uncommon. The same is true, but the threshold for renal excretion is not low, and the incidence of upward alpha-HNF 4 mutations in cases with a solid clinical panel for alpha HNF 1 77, but not confirmed by genetic sequencing, should be considered. The disease is also susceptible to sulfonylureas:
MODY4 mutation in the MODY6 gene, IPF1, with a mutation in MODY7, NeuroD1, is characterized by a carboxy sterilipase mutation, which is not typical: MODY2. The increase in fasting blood glucose in 100 to 150 mg/dl is uncommon in children and adolescents. The incidence of this condition is usually considered to be type 1 or 2 diabetes, but a large percentage of the above patients are heterozygote individuals, the glucokinase mutation mutations 78 (figures 1 and 2).
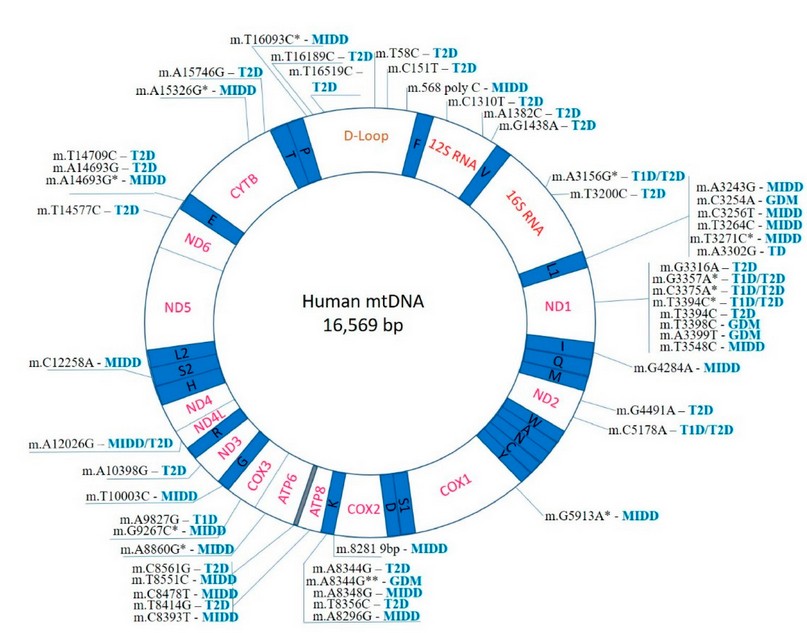
Figure 1. Mutations in the human mitochondria and diabetes (Biorender Program).
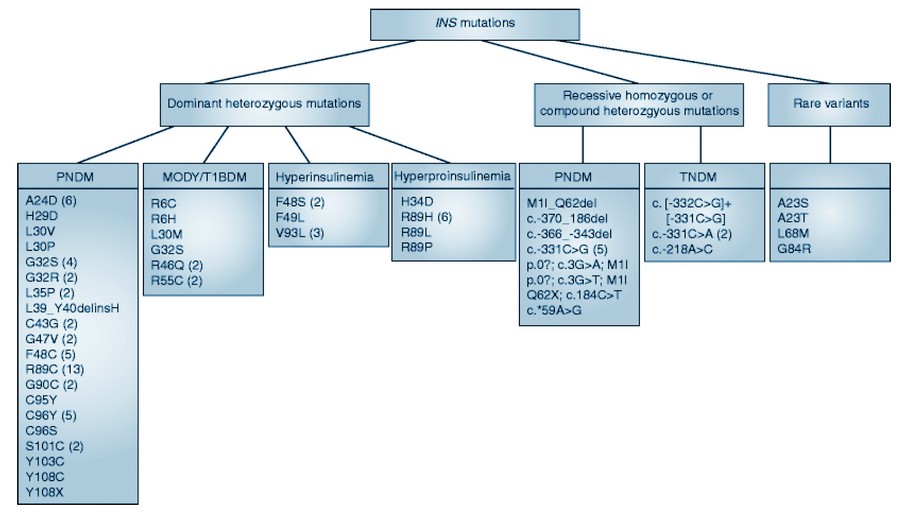
Figure 2. Pediatrics diabetes and insulin mutations (Biorender Program).
Identifying the genetic mutations underlying early-onset diabetes is critical for determining the specific diabetes subtype, allowing for proper treatment and assessment of recurrence risk in offspring. Given the disease’s great genetic and clinical heterogeneity, high-throughput sequencing may provide additional diagnostic insight if Sanger sequencing is ineffective 79. In one survey, 102 genes were re-sequenced in 30 patients who tested negative for mutations in the GCK, GCK, HNF1α, HNF4α, HNF1β, and IPF1 genes using Sanger sequencing. Undetermined mutations in the RFX6 gene were discovered in three patients, and rare variants in the WFS1 and ABCC8 genes were discovered in two of them 23. All of the patients responded favorably to dipeptidyl peptidase-4 (DPP4) inhibitors. According to their findings, next-generation sequencing (NGS) is a susceptible method for identifying variants in new diabetes-causing genes. This path may help to understand the molecular etiology of diabetes and provide more personalized treatment for each genetic subtype 80.
Diabetes mellitus is linked to several natural mutations in the human insulin gene. Wakayama, Los Angeles, and Chicago mutant molecules were evaluated using molecular docking and molecular dynamics (MD) to investigate mechanisms of deprived binding affinity for insulin receptors (IR) 23. Insulin Wakayama, is a variant in which valine at position A3 is substituted by leucine. In contrast, in insulin, Los Angeles and Chicago, phenylalanine at positions B24 and B25 are replaced by serine and leucine, respectively 81. These mutations cause significant changes in IR binding affinity. The molecular docking was done using the ZDOCK server, and the MD study was done using AMBER 14. MD was also performed using the previously published crystal structure of IR bound to natural insulin. In detail, the binding interactions and MD trajectories explained the critical factors for deprived binding to the IR. When valine was replaced by leucine, the surface area around position A3 increased. In contrast, at positions B24 and B25, the aromatic amino acid phenylalanine was replaced by non-aromatic serine and leucine, which may be responsible for fewer binding interactions at the IR binding site, leading to complex instability. The standard mode analysis, rmsd trajectories, and fluctuation prediction in the MD simulation indicated instability of complexes with mutant insulin in the order of native insulin Chicago insulin, los Angeles insulin Wakayama molecules, which corresponds to the biological evidence of the mutant insulins’ differing affinities for the IR 81,82.
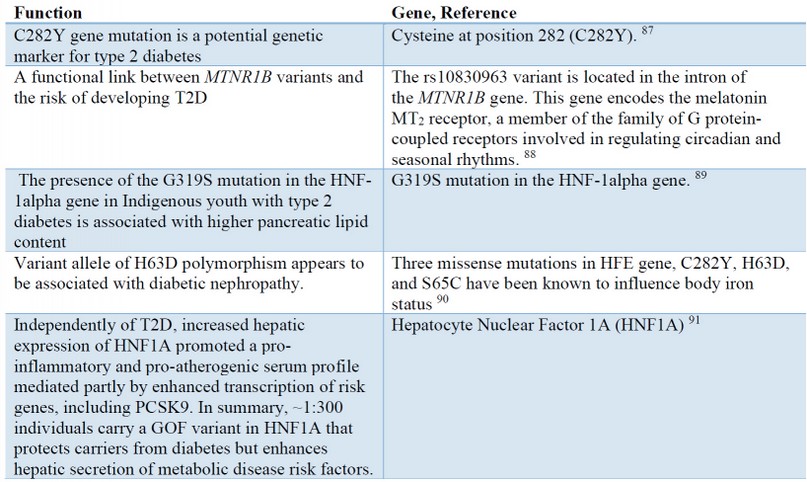
Table 1. Major mutations in the T2DM
Direct sequencing was used to screen the INS gene. A mixed-meal test was performed on the probands and their affected relatives. I-TASSER was used to model mutation predictions, which were then visualized using Swiss-Pdb Viewer 83. In three generations of patients with clinically distinct diabetes, a novel heterozygous frameshift mutation p.Gln78fs in the INS gene was discovered. The single nucleotide deletion (c.233delA) is predicted to change and lengthen the amino acid sequence, resulting in aberrant proinsulin lacking native C-peptide and A-chain structures. The heterozygous mutation c.188-31G>A within the terminal intron was discovered in the second family. The mother and her daughter were misdiagnosed with type 1 diabetes when they were 6 and 2 years old, respectively. This finding contrasts with the previously reported case of a carrier of the same mutation diagnosed with permanent neonatal diabetes. We discovered a new coding frameshift mutation and an intronic mutation in the INS gene that cause childhood diabetes. INS mutations can cause a variety of phenotypes, implying that additional mechanisms are at work in the pathogenesis and clinical manifestation of diabetes 84.
The glutamine fructose 6-phosphate aminotransferase (GFPT2) gene, which is located on the long arm of chromosome 5, has recently been linked to type 2 diabetes. The TT genotype was linked to the disease in one study. Compared to the control, the nitric oxide synthase gene (eNOS) mutation in Glu 298 Asp was associated with type 2 diabetes 23. Mendelian diabetes develops as a result of molecular mechanisms that modulate cell pathophysiology. As a result, Class IIa histone deacetylases (HDAC4, 5, 7, and 9) regulate pancreatic endocrine cell activity and glucose homeostasis in mammals. Sanger sequencing on mouse pancreatic cell lines (Min6 and SJ cells) identified an HDAC4 variant (p.His227Arg) as a disease determinant. However, two other variants, p.Asp234Asn and p.Glu374Lys were also found in non-autoimmune diabetes 85.
CONCLUSION
Diabetes mellitus (DM) is caused by a lack of ability to produce or secrete insulin or by insulin resistance; thus, insulin production impairment causes the disease. Individuals can control some factors that contribute to the onset of the disease, such as weight, impulsivity, blood pressure, and lipids. A single genetic mutation affects about 3% of diabetic patients. Interestingly, more than 20 genes are involved in the function of beta cells. Improved therapy, better prediction of disease prognosis and progression, genetic counseling, and possibly prevention are all benefits of gene diagnosis.
Alpha HNF1 mutations in the early stages may respond to the regimen. Still, most patients need it because they control their blood glucose and will be subject to microvascular or macrovascular complications. In cases where insulin does not control sugar, using low-dose sulfonylureas will be beneficial and lower 4 times the glucose metabolism of metformin. These patients are susceptible to sulfonylureas and may be treated for years in case of no blood glucose attack complications. The drug will start at one-fourth of the adult dose: MODY1. It is caused by a mutation in the alpha-HNF 4 gene and is relatively uncommon. The same is true, but the threshold for renal excretion is not low. The incidence of upward alpha-HNF 4 mutations in cases with a robust clinical panel for alpha-HNF 1 but not confirmed by genetic sequencing should be considered. The disease is also susceptible to sulfonylureas: MODY4 with a mutation in the MODY6 gene, IPF1, with a mutation in MODY7, NeuroD1 is characterized by a carboxy sterilipase mutation, which is not typical: MODY2. The increase in fasting blood glucose in 100 to 150 mg/dl is uncommon in children and adolescents. The incidence of this condition is usually considered to be type 1 or 2 diabetes, but a large percentage of the above patients are heterozygote individuals, the glucokinase mutations. Other mutations associated with T2DM include rare variants in the WFS1 and ABCC8 genes, insulin receptor (IR), fructose 6-phosphate aminotransferase (GFPT2), nitric oxide synthase (eNOS), and mouse pancreatic cell lines (Min6 and SJ cells) revealed HDAC4 variant (p.His227Arg).
Conflict of interest. None
Acknowledgments. The authors wrote this study.
REFERENCES
1. McIntyre HD, Catalano P, Zhang C, Desoye G, Mathiesen ER, Damm P. Gestational diabetes mellitus. Nature reviews Disease primers 2019,5:1-19.
2. Sapra A, Bhandari P. Diabetes mellitus. 2019.
3. Oguntibeju OO. Type 2 diabetes mellitus, oxidative stress and inflammation: examining the links. International journal of physiology, pathophysiology and pharmacology 2019,11:45.
4. Johns EC, Denison FC, Norman JE, Reynolds RM. Gestational diabetes mellitus: mechanisms, treatment, and complications. Trends in Endocrinology & Metabolism 2018,29:743-754.
5. Biessels GJ, Despa F. Cognitive decline and dementia in diabetes mellitus: mechanisms and clinical implications. Nature Reviews Endocrinology 2018,14:591-604.
6. Glovaci D, Fan W, Wong ND. Epidemiology of diabetes mellitus and cardiovascular disease. Current cardiology reports 2019,21:1-8.
7. Crowshoe L, Dannenbaum D, Green M, Henderson R, Hayward MN, Toth E. Type 2 diabetes and Indigenous peoples. Canadian Journal of Diabetes 2018,42:S296-S306.
8. Zheng Y, Ley SH, Hu FB. Global aetiology and epidemiology of type 2 diabetes mellitus and its complications. Nature Reviews Endocrinology 2018,14:88-98.
9. Powers MA, Bardsley JK, Cypress M, Funnell MM, Harms D, Hess-Fischl A, et al. Diabetes self-management education and support in adults with type 2 diabetes: a consensus report of the American Diabetes Association, the Association of Diabetes Care & Education Specialists, the Academy of Nutrition and Dietetics, the American Academy of Family Physicians, the American Academy of PAs, the American Association of Nurse Practitioners, and the American Pharmacists Association. Diabetes Care 2020,43:1636-1649.
10. Delaney KZ, Santosa S. Sex differences in regional adipose tissue depots pose different threats for the development of Type 2 diabetes in males and females. Obesity Reviews 2022:e13393.
11. Verhagen C, Janssen J, Biessels GJ, Johansen OE, Exalto LG. Females with type 2 diabetes are at higher risk for accelerated cognitive decline than males: CAROLINA-COGNITION study. Nutrition, Metabolism and Cardiovascular Diseases 2022,32:355-364.
12. Xiang AS, Szwarcbard N, Gasevic D, Earnest A, Pease A, Andrikopoulos S, et al. Trends in glycaemic control and drug use in males and females with type 2 diabetes: Results of the Australian National Diabetes Audit from 2013 to 2019. Diabetes, Obesity and Metabolism 2021,23:2603-2613.
13. Plows JF, Stanley JL, Baker PN, Reynolds CM, Vickers MH. The pathophysiology of gestational diabetes mellitus. International journal of molecular sciences 2018,19:3342.
14. Davenport MH, Ruchat S-M, Poitras VJ, Garcia AJ, Gray CE, Barrowman N, et al. Prenatal exercise for the prevention of gestational diabetes mellitus and hypertensive disorders of pregnancy: a systematic review and meta-analysis. British journal of sports medicine 2018,52:1367-1375.
15. Saravanan P, Magee LA, Banerjee A, Coleman MA, Von Dadelszen P, Denison F, et al. Gestational diabetes: opportunities for improving maternal and child health. The Lancet Diabetes & Endocrinology 2020,8:793-800.
16. Lowe WL, Scholtens DM, Kuang A, Linder B, Lawrence JM, Lebenthal Y, et al. Hyperglycemia and Adverse Pregnancy Outcome Follow-up Study (HAPO FUS): maternal gestational diabetes mellitus and childhood glucose metabolism. Diabetes care 2019,42:372-380.
17. Redondo MJ, Steck AK, Pugliese A. Genetics of type 1 diabetes. Pediatric diabetes 2018,19:346-353.
18. Morelli MB, Wang X, Santulli G. Functional role of gut microbiota and PCSK9 in the pathogenesis of diabetes mellitus and cardiovascular disease. Atherosclerosis 2019,289:176.
19. Oliveira SC, Neves JS, Pérez A, Carvalho D. Maturity-onset diabetes of the young: from a molecular basis perspective toward the clinical phenotype and proper management. Endocrinologia, diabetes y nutricion 2020,67:137-147.
20. Bobiş O, Dezmirean DS, Moise AR. Honey and diabetes: the importance of natural simple sugars in diet for preventing and treating different type of diabetes. Oxidative medicine and cellular longevity 2018,2018.
21. Zubkova NA, Burumkulova FF, Ulyatovskaya VI, Petrukhin VA, Plechanova MA, Panov AE, et al. Birth weight and length in offsprings of mothers with gestational diabetes mellitus due to mutations in GCK gene. Diabetes mellitus 2018,21:92-98.
22. McCarthy M. Approaches to determining the genetic basis of non-insulin-dependent diabetes mellitus. In: Genetics of Common Diseases: Garland Science; 2020. pp. 91-128.
23. Alghanimi YK. The association of GFPT2 and Glu 298 Asp gene variations to the type 2 diabetes. Gene Reports 2020,19:100579.
24. Barbetti F, D’Annunzio G. Genetic causes and treatment of neonatal diabetes and early childhood diabetes. Best Practice & Research Clinical Endocrinology & Metabolism 2018,32:575-591.
25. Calcaterra V, De Silvestri A, Schneider L, Acunzo M, Vittoni V, Meraviglia G, et al. Acanthosis Nigricans in Children and Adolescents with Type 1 Diabetes or Obesity: The Potential Interplay Role between Insulin Resistance and Excess Weight. Children 2021,8:710.
26. Afrooz F, Afsana F, Amin MF, Mustafaa SJ, Rouf RSB, Hossain KN, et al. Rabson-Mendenhall syndrome: a rare case of severe insulin resistance. BIRDEM Medical Journal 2022,12:83-87.
27. Banerjee A, Singh S, Prasad SK, Kumar S, Banerjee O, Seal T, et al. Protective efficacy of Tinospora sinensis against streptozotocin induced pancreatic islet cell injuries of diabetic rats and its correlation to its phytochemical profiles. Journal of ethnopharmacology 2020,248:112356.
28. Malla A, Mukherjee K, Mandal M, Mukherjee A, Sur R, Gupta S. An Insight to the Toxic Effect of Sulfamerazine on Porcine Pancreatic Amylase and Lactate Dehydrogenase Activity: An In Vitro Study. Current Chemical Biology 2021,15:171-181.
29. Kostov K. Effects of magnesium deficiency on mechanisms of insulin resistance in type 2 diabetes: focusing on the processes of insulin secretion and signaling. International journal of molecular sciences 2019,20:1351.
30. Barbot M, Ceccato F, Scaroni C. Diabetes mellitus secondary to Cushing’s disease. Frontiers in endocrinology 2018,9:284.
31. Lombardi A, Tsomos E, Hammerstad SS, Tomer Y. Interferon alpha: the key trigger of type 1 diabetes. Journal of autoimmunity 2018,94:7-15.
32. Roth J, Müller N, Kuniss N, Wolf G, Müller UA. Association between glycaemic control and the intake of thiazide diuretics, beta blockers and levothyroxine in people without diabetes. Experimental and Clinical Endocrinology & Diabetes 2021,129:443-448.
33. Magee MJ, Salindri AD, Kornfeld H, Singhal A. Reduced prevalence of latent tuberculosis infection in diabetes patients using metformin and statins. European respiratory journal 2019,53.
34. Takeno A, Kanazawa I, Morita M, Takedani K, Miyake H, Yamamoto M, et al. A case report of fulminant type 1 diabetes mellitus associated with drug-induced hypersensitivity syndrome in an elderly patient with coxsackie B4 virus infection and human leukocyte antigen-A24 haplotype. Endocrine journal 2018,65:129-132.
35. Shi D, Motamed M, Mejía‐Benítez A, Li L, Lin E, Budhram D, et al. Genetic syndromes with diabetes: a systematic review. Obesity Reviews 2021,22:e13303.
36. Brás IC, König A, Outeiro TF. Glycation in Huntington’s disease: a possible modifier and target for intervention. Journal of Huntington’s disease 2019,8:245-256.
37. Li Y, Teng D, Shi X, Qin G, Qin Y, Quan H, et al. prevalence of diabetes recorded in mainland China using 2018 diagnostic criteria from the American Diabetes Association: national cross sectional study. Bmj 2020,369.
38. Buzzetti R, Tuomi T, Mauricio D, Pietropaolo M, Zhou Z, Pozzilli P, et al. Management of latent autoimmune diabetes in adults: a consensus statement from an international expert panel. Diabetes 2020,69:2037-2047.
39. Bingley PJ, Wherrett DK, Shultz A, Rafkin LE, Atkinson MA, Greenbaum CJ. Type 1 Diabetes TrialNet: a multifaceted approach to bringing disease-modifying therapy to clinical use in type 1 diabetes. Diabetes Care 2018,41:653-661.
40. Forouhi NG, Wareham NJ. Epidemiology of diabetes. Medicine 2019,47:22-27.
41. Madhusudhanan J, Suresh G, Devanathan V. Neurodegeneration in type 2 diabetes: Alzheimer’s as a case study. Brain and behavior 2020,10:e01577.
42. Gupta S, Singhal NK, Ganesh S, Sandhir R. Extending arms of insulin resistance from diabetes to Alzheimer’s disease: identification of potential therapeutic targets. CNS & Neurological Disorders-Drug Targets (Formerly Current Drug Targets-CNS & Neurological Disorders) 2019,18:172-184.
43. Klimova B, Kuca K, Maresova P. Global view on Alzheimer’s Disease and Diabetes Mellitus: threats, risks and treatment Alzheimer’s Disease and Diabetes Mellitus. Current Alzheimer Research 2018,15:1277-1282.
44. Du YT, Rayner CK, Jones KL, Talley NJ, Horowitz M. Gastrointestinal symptoms in diabetes: prevalence, assessment, pathogenesis, and management. Diabetes care 2018,41:627-637.
45. Strain WD, Paldánius P. Diabetes, cardiovascular disease and the microcirculation. Cardiovascular diabetology 2018,17:1-10.
46. Bellou V, Belbasis L, Tzoulaki I, Evangelou E. Risk factors for type 2 diabetes mellitus: an exposure-wide umbrella review of meta-analyses. PloS one 2018,13:e0194127.
47. Xu T, Hanson LLM, Lange T, Starkopf L, Westerlund H, Madsen IE, et al. Workplace bullying and violence as risk factors for type 2 diabetes: a multicohort study and meta-analysis. Diabetologia 2018,61:75-83.
48. Alperet DJ, Rebello SA, Khoo EY-H, Tay Z, Seah SS-Y, Tai B-C, et al. The effect of coffee consumption on insulin sensitivity and other biological risk factors for type 2 diabetes: a randomized placebo-controlled trial. The American journal of clinical nutrition 2020,111:448-458.
49. Regmi D, Al-Shamsi S, Govender RD, Al Kaabi J. Incidence and risk factors of type 2 diabetes mellitus in an overweight and obese population: a long-term retrospective cohort study from a Gulf state. BMJ open 2020,10:e035813.
50. Ghaemi F, Fateh A, Sepahy AA, Zangeneh M, Ghanei M, Siadat SD. Intestinal microbiota composition in iranian diabetic, pre-diabetic and healthy individuals. Journal of Diabetes & Metabolic Disorders 2020,19:1199-1203.
51. Zheng X, Chen T, Zhao A, Ning Z, Kuang J, Wang S, et al. Hyocholic acid species as novel biomarkers for metabolic disorders. Nature communications 2021,12:1-11.
52. Kattamis C, Ladis V, Skafida M, Iacovidou N, Theodoridis C. The different patterns of insulin response during oral glucose tolerance test (OGTT) in transfused young patients with β-thalassemia. Acta Bio Medica: Atenei Parmensis 2021,92.
53. Zhang M, Ding R, Wang Z, Zhang J, Liu J, Wang J. Altered Peak C-peptide and Fasting Blood Glucose in Children with Autism Spectrum Disorder. Journal of Diabetes and Clinical Research 2019,1.
54. Bjornstad P, Hughan K, Kelsey MM, Shah AS, Lynch J, Nehus E, et al. Effect of surgical versus medical therapy on diabetic kidney disease over 5 years in severely obese adolescents with type 2 diabetes. Diabetes care 2020,43:187-195.
55. YOU S, KANG M. A Study on Methods to Prevent Pima Indians Diabetes using SVM. Korea Journal of Artificial Intelligence 2020,8:7-10.
56. Chukwuma CI, Matsabisa MG, Erukainure OL, Ibeji CU, Islam MS. D-mannitol modulates glucose uptake ex vivo; suppresses intestinal glucose absorption in normal and type 2 diabetic rats. Food Bioscience 2019,29:30-36.
57. Nair GG, Tzanakakis ES, Hebrok M. Emerging routes to the generation of functional β-cells for diabetes mellitus cell therapy. Nature Reviews Endocrinology 2020:1-13.
58. Onodera T, Scherer PE. From friend to foe: Pro-apoptotic action of nuclear ARC in diabetes. Developmental cell 2021,56:717-718.
59. Moin ASM, Butler AE. Alterations in beta cell identity in type 1 and type 2 diabetes. Current diabetes reports 2019,19:1-12.
60. Iyikesici MS. Feasibility study of metabolically supported chemotherapy with weekly carboplatin/paclitaxel combined with ketogenic diet, hyperthermia and hyperbaric oxygen therapy in metastatic non-small cell lung cancer. International Journal of Hyperthermia 2019,36:445-454.
61. Zhang M, Lin S, Yuan X, Lin Z, Huang Z. HLA-DQB1 and HLA-DRB1 Variants Confer Susceptibility to Latent Autoimmune Diabetes in Adults: Relative Predispositional Effects among Allele Groups. Genes 2019,10:710.
62. De Franco E, Saint‐Martin C, Brusgaard K, Knight Johnson AE, Aguilar‐Bryan L, Bowman P, et al. Update of variants identified in the pancreatic β‐cell KATP channel genes KCNJ11 and ABCC8 in individuals with congenital hyperinsulinism and diabetes. Human mutation 2020,41:884-905.
63. Touati A, Errea-Dorronsoro J, Nouri S, Halleb Y, Pereda A, Mahdhaoui N, et al. Transient neonatal diabetes mellitus and hypomethylation at additional imprinted loci: novel ZFP57 mutation and review on the literature. Acta diabetologica 2019,56:301-307.
64. Farhadova S, Gomez-Velazquez M, Feil R. Stability and lability of parental methylation imprints in development and disease. Genes 2019,10:999.
65. Bonfanti R, Iafusco D, Rabbone I, Diedenhofen G, Bizzarri C, Patera PI, et al. Differences between transient neonatal diabetes mellitus subtypes can guide diagnosis and therapy. European journal of endocrinology 2021,184:575-585.
66. Evliyaoğlu O, Ercan O, Ataloğlu E, Zübarioğlu Ü, Özcabı B, Dağdeviren A, et al. Neonatal diabetes: two cases with isolated pancreas agenesis due to homozygous PTF1A enhancer mutations and one with developmental delay, epilepsy, and neonatal diabetes syndrome due to KCNJ11 mutation. Journal of clinical research in pediatric endocrinology 2018,10:168.
67. Misra S, Owen KR. Genetics of monogenic diabetes: present clinical challenges. Current diabetes reports 2018,18:1-11.
68. Segovia-Ortí R, de los Monteros Aliaga NE, Lumbreras J, de Sotto-Esteban D, Rodrigo MD. Renal Dysplasia and Precocious Diabetes Onset in Microcephalic Osteodysplastic Primordial Dwarfism Type II Syndrome: A Case Report. Journal of Pediatric Genetics 2020.
69. Ovsyannikova A, Rymar O, Ivanoshchuk D, Mikhailova SV, Shakhtshneider E, Orlov P, et al. A case of maturity onset diabetes of the young (MODY3) in a family with a novel HNF1A gene mutation in five generations. Diabetes therapy 2018,9:413-420.
70. Buchanan K, Mehdi AM, Hughes I, Cotterill A, Le Cao KA, Thomas R, et al. An improved clinical model to predict stimulated C‐peptide in children with recent‐onset type 1 diabetes. Pediatric diabetes 2019,20:166-171.
71. Lai Y, Qi J, Tao X, Huang K, Yan S, Chen M, et al. Associations of grandparental diabetes mellitus with grandchild BMI status. BMC public health 2019,19:1-8.
72. Granados A, Chan CL, Ode KL, Moheet A, Moran A, Holl R. Cystic fibrosis related diabetes: Pathophysiology, screening and diagnosis. Journal of Cystic Fibrosis 2019,18:S3-S9.
73. Christensen AS, Hædersdal S, Støy J, Storgaard H, Kampmann U, Forman JL, et al. Efficacy and safety of glimepiride with or without linagliptin treatment in patients with HNF1A diabetes (maturity-onset diabetes of the young type 3): a randomized, double-blinded, placebo-controlled, crossover trial (GLIMLINA). Diabetes Care 2020,43:2025-2033.
74. Støy J, De Franco E, Ye H, Park S-Y, Bell GI, Hattersley AT. In celebration of a century with insulin–Update of insulin gene mutations in diabetes. Molecular metabolism 2021,52:101280.
75. Cardenas-Diaz FL, Osorio-Quintero C, Diaz-Miranda MA, Kishore S, Leavens K, Jobaliya C, et al. Modeling monogenic diabetes using human ESCs reveals developmental and metabolic deficiencies caused by mutations in HNF1A. Cell stem cell 2019,25:273-289. e275.
76. Urakami T. Maturity-onset diabetes of the young (MODY): current perspectives on diagnosis and treatment. Diabetes, metabolic syndrome and obesity: targets and therapy 2019,12:1047.
77. Monsonego S, Clark H, Karovitch A, O’Meara P, Shaw T, Malcolm J. Management and outcomes of maturity-onset diabetes of the young in pregnancy. Canadian journal of diabetes 2019,43:647-654.
78. Kumar A, Kumar A, Samadarshi S, Manrai M, Tevatia MS, Dawra S. Unknown presentation of a rare genetic disorder: Monogenic diabetes in young type 4 presenting with hepatic cysts and procoagulant state. Medical Journal Armed Forces India 2022.
79. Firdous P, Nissar K, Ali S, Ganai BA, Shabir U, Hassan T, et al. Genetic testing of maturity-onset diabetes of the young current status and future perspectives. Frontiers in endocrinology 2018,9:253.
80. Saberzadeh-Ardestani B, Karamzadeh R, Basiri M, Hajizadeh-Saffar E, Farhadi A, Shapiro AJ, et al. Type 1 diabetes mellitus: cellular and molecular pathophysiology at a glance. Cell Journal (Yakhteh) 2018,20:294.
81. Ito S, Torii Y, Chikamatsu S, Harada T, Yamaguchi S, Ogata S, et al. Oral Coadministration of Zn-Insulin with d-Form Small Intestine-Permeable Cyclic Peptide Enhances Its Blood Glucose-Lowering Effect in Mice. Molecular Pharmaceutics 2021,18:1593-1603.
82. Yu F, Wang Y, Teng Y, Yang S, He Y, Zhang Z, et al. Interaction and Inhibition of a Ganoderma lucidum Proteoglycan on PTP1B Activity for Anti-diabetes. ACS omega 2021,6:29804-29813.
83. Kannan N. A system-level approach to investigate alloxan-induced toxicity in microtubule-binding protein to lead type 2 diabetes mellitus. Molecular Diversity 2020.
84. Musthaffa Y, Hamilton‐Williams EE, Nel HJ, Bergot AS, Mehdi AM, Harris M, et al. Proinsulin‐specific T‐cell responses correlate with estimated c‐peptide and predict partial remission duration in type 1 diabetes. Clinical & Translational Immunology 2021,10:e1315.
85. Gong M, Yu Y, Liang L, Vuralli D, Froehler S, Kuehnen P, et al. HDAC4 mutations cause diabetes and induce β‐cell FoxO1 nuclear exclusion. Molecular genetics & genomic medicine 2019,7:e602.
86. Banoon SR, Salih TS, Ghasemian A. Genetic Mutations and Major Human Disorders: A Review. Egyptian Journal of Chemistry. 2022,65: 571-589.
87. Kwan T, Leber B, Ahuja S, Carter R, Gerstein HC. Patients with type 2 diabetes have a high frequency of the C282Y mutation of the hemochromatosis gene. Clinical and investigative medicine. 1998 Dec 1;21(6):251.
88. Karamitri A, Vincens M, Chen M, Jockers R. Implication des mutations du récepteur de la mélatonine MT2 dans la survenue du diabète de type 2. médecine/sciences. 2013 Aug 1;29(8-9):778-84.
89. Wicklow BA, Griffith AT, Dumontet JN, Venugopal N, Ryner LN, McGavock JM. Pancreatic lipid content is not associated with beta cell dysfunction in youth-onset type 2 diabetes. Canadian journal of diabetes. 2015 Oct 1;39(5):398-404.
90. Muhammad JS, Islam N, Mehboobali N, Iqbal K, Azam I, Iqbal MP. Lack of association of HFE gene polymorphism with high body iron status in Pakistani patients with type 2 diabetes mellitus. Journal of the Pakistan Medical Association. 2021;71(2):608-13.
91. DeForest N, Kavitha B, Hu S, Isaac R, Wang M, Du X, Saldanha CD, Gylys J, Abagyan R, Najmi L, Mohan V. Human gain-of-function variants in HNF1A confer protection from diabetes but independently increase hepatic secretion of multiple cardiovascular disease risk factors. medRxiv. 2022 January 1.
Received: September 22, 2022 / Accepted: October 18, 2022 / Published:15 November 2022
Citation: Banoon S R, Alfathi M Y, Shokouhi Mostafavi S K, Ghasemian A. Predominant genetic mutations leading to or predisposing diabetes progress: A Review. Revis Bionatura 2022;7(4) 66. http://dx.doi.org/10.21931/RB/2022.07.04.66