Vol 8 No 3 2023 – 37
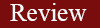
A look into Honduran biomass: facts, uses and potential applications
David Bulnes 1
, Sofía Melgar 1
, Edgard Vega 1
, Aaron Rubio 1
, Andrea Espinal 1
, Diana G. Velásquez-Tinoco 1
, Gloria Díaz Reyes 1
, Evelina D. Estrada-López 1
, Oscar V. Ortiz-Hernández 1,*









1 Universidad Nacional Autónoma de Honduras /Tegucigalpa/Honduras; david.altamirano@unah.hn, sofia.melgar@unah.hn, edgard.vega@unah.hn, rodolfo.rubio@unah.hn. acespinal@unah.hn, diana.velasquez@unah.edu.hn, gloria.diaz@unah.edu.hn, evelina.estrada@unah.edu.hn, oscar.ortiz@unah.edu.hn.
* Correspondence: oscar.ortiz@unah.edu.hn; Tel.: +504 3148-0879
Available from: http://dx.doi.org/10.21931/RB/2023.08.03.37
ABSTRACT
Agro-industrial biomasses are materials that come from the transformation of sources of vegetable or animal origin generally used for energy processes. This bibliographic review describes five economic interest biomasses in Honduras: coffee, African palm, sugarcane, shrimp, and cashew. A general description of each biomass is made, contextualizing the economic importance in the country, a brief description of the production process, current uses, and possible future applications.
Keywords: biorefinery, biotechnology, nanomaterials, biofuel, agro-industrial waste, environment.
INTRODUCTION
In many countries, the agro-industry represents an essential impact on the global economy, generating foreign exchange and employment. Honduras is the primary sector of economic development, followed by the manufacturing, agricultural, and mining sectors.1
However, these beneficial practices for society generate considerable amounts of waste, which in many cases represent environmental problems that are difficult to manage. These residues have important physicochemical characteristics that could enhance the circular economy and minimize ecological issues.
Until December 2021, agro-industrial activity accounted for 53% of total export earnings, with coffee being the main product in this agro-industrial category, followed by palm and shrimp. However, according to the Central Bank, there was evidence of a deficit in the import-export ratio, mainly due to imports of raw materials, consumer goods, and fuel. 1
To improve this deficit, Honduras must export more products or decrease imports. A window of opportunity is the use of waste generated in agribusiness. Depending on their origin, these residues contain essential chemical compounds that can be extracted, transformed, and used in many industrial applications to create products or raw materials that can no longer be imported as they are generated locally.
Biomass from agribusiness has various possibilities and can be the basis for developing a biorefinery in Honduras that generates energy and chemical products. It is a cleaner and more environmentally friendly alternative to replace fuels and chemical products from non-renewable sources.
Currently, in Honduras, most of the biomass generated in agribusiness is used for energy production, taking advantage of its calorific value. For example, biomass is directly burned, as occurs with parchment or coffee husk, palm fiber or shell, the sugarcane bagasse, and the cashew nutshell. On the other hand, biomasses that do not have a direct application in energy, as is the case of shrimp waste, are used for animal feed production.
Based on the literature review, this work is the first to evaluate the current uses and other potential applications of biomass generated in Honduras, focusing on those from coffee, palm, sugarcane, shrimp, and cashews, presented in Figure 1.
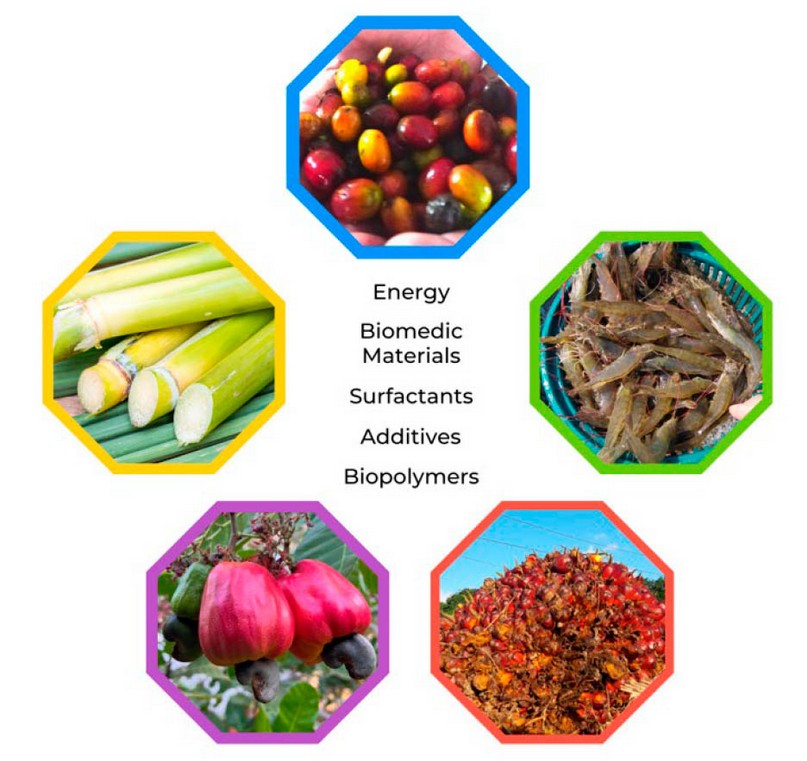
Figure 1. The primary biomass generated in Honduras are coffee, palm, sugarcane, shrimp, and cashew.
Coffee
The coffee bean is prepared as one of the most popular drinks worldwide. This is due to people associating its consumption with «energetic» benefits that will help them do daily activities. With psychostimulant substances aid, such as caffeine, people hope to lighten the struggle of daily activities.2,3
Most coffee bean production comes from two species: Coffea arabica (Arabica) and Coffea canephora (Robusta). Both species have gone through multiple analytical techniques that have revealed their differences.4–6 The economic importance of coffee among the producing and consuming countries has led to profound studies interested in differentiating varieties through plant genetics, sensory properties, and cup quality.7,8
According to the International Coffee Organization (OIC), the worldwide production for the coffee year 2020/2021 was 168.88 million 60 kg bags, representing a 0.1% decrease from last year’s production. Nevertheless, the Arabica production was 99.26 million bags, representing a 2.2% increase from the 2019/2020 production. The general decrease in production is due to the Robusta species.9
The OIC states that ten countries represent 89% of worldwide production. Brazil, Vietnam, and Colombia dominate the list. Indonesia, Ethiopia, Honduras, Uganda, India, Mexico, and Peru follow these countries. The mentioned countries sustain global coffee consumption. In the ten years before 2020, coffee consumption increased by 1.9% on average. In 2022, an increase of 3.3% was expected.9,10
According to the OIC and the Honduran Coffee Institute (IHCAFE for its Spanish acronym), Honduras takes sixth place among the highest coffee-producing countries. According to the OIC reports, Honduras represented 3% of the global coffee production in 2020/2021. Figure 2 shows the Honduran production in millions of 46 kg bags according to IHCAFE through the coffee years of 2016/2017 to 2020/2021; as shown in Figure 2, a slight increase in production is appreciated in the periods of 2016/2017 to 2017/2018. On the other hand, a decrease in production was reported from 2017/2018 to 2020/2021. This decrease caused Honduras to lose fifth place in the list, going down to sixth place.11
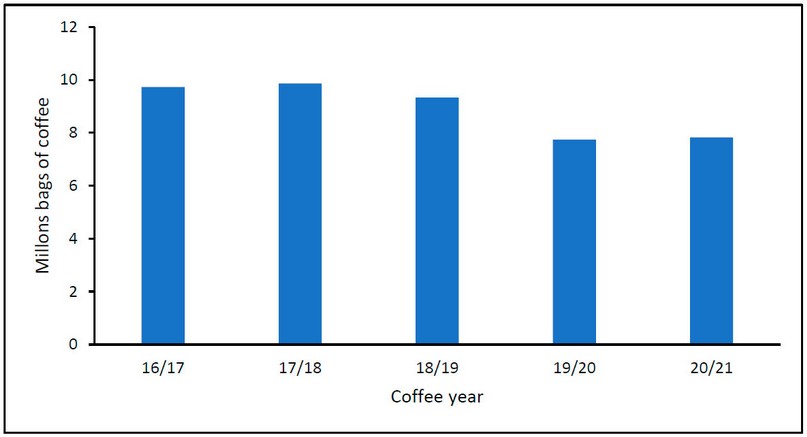
Figure 2. Coffee production in Honduras in millions of 46 kg bags in the harvest from 2016 to 2021.
The coffee bean is the most important agricultural product in Honduran exports. This is due to its impact as a source of foreign exchange. The National Statistics Institute (INE for its Spanish acronym) reported 868 million dollars in exportations for 2020, a decrease from previous years. In 2017, a historical amount of 1.29 billion dollars in coffee bean exports was recorded.12
Temperature, altitude, and precipitation affect the Honduran coffee bean production. The optimal growing conditions for a 100% Honduran Arabica type coffee are temperatures ranging from 14 to 26 °C, altitudes from 400 to 1200 meters above sea level for tropical regions, and annual 1000-2700 mm precipitations.13–15
The coffee bean comes from the coffee fruit, locally known as the «coffee grape» or the «coffee cherry.» 16 The fruit’s morphology is shown in Figure 3. It consists of an exocarp, the outer skin, which changes color from green to red due to its growth. This changing color is a significant parameter to consider in its harvest. The mesocarp is a sticky layer abundant in carbohydrates and derivatives such as pectin. The mesocarp consists of a pulp and mucilage. The endocarp, known as parchment, is a thin yellowish layer that protects the endosperm or the coffee bean, which has a layer known as the silver skin.8
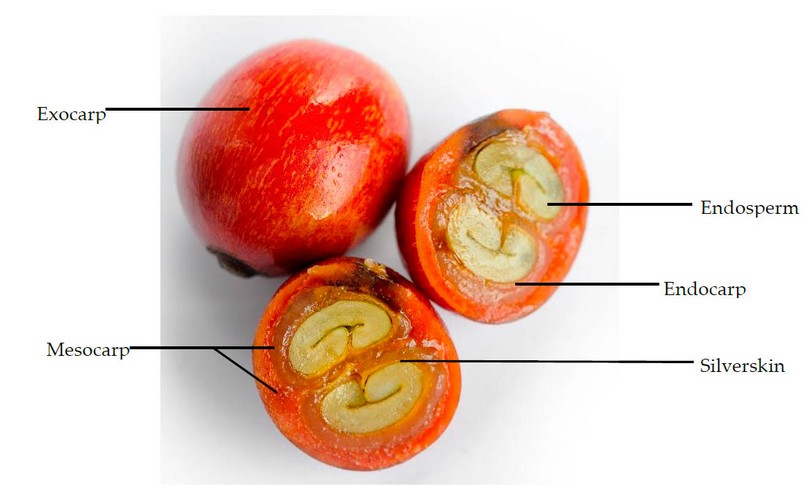
Figure 3. Morphology of the coffee fruit.
The commercially important part, the coffee cherry, is agro-industrially processed to obtain the coffee bean. These operations are known as coffee processing. This process follows steps ranging from the coffee fruit reception to the drying without parchment, known internationally as green coffee beans.17 The processing can be done in different ways, in a dry or wet way. Dry processed coffee is the oldest and easiest way to obtain the grain, which consists of a single sun-drying stage of the coffee cherry immediately after its harvest. Through this method, we get natural or sun-dried coffee. The washed coffee is obtained through the wet method, using large amounts of water. Wet coffee processing consists of two stages: the wet and dry stages. The wet stage consists of a series of steps going from the fruit reception, de-pulping, demucilaging (fermentation), washing, classification, and drying until we get a seed with 12% humidity. Once the ideal humidity levels are reached, it begins with the dry stage, starting with storage, threshing, classifying, tasting, and packing. 90% of Honduran-produced coffee is wet-processed and is destined for exportation. The other 10% is dry processed and is used for national consumption.15 Coffee processing is a crucial stage that uses the big differences in the coffee beans’ anatomy, chemical composition, and humidity, affecting the final product’s quality in its sensory properties and the number of generated by-products.18
With the dry processing method, only one by-product is generated by mechanical threshing after drying. The by-product consists of the coffee husk of the skin, pulp, mucilage, and parchment. All of this represents about 45% of the fruit.8,19 On the other hand, with the wet processing method, the skin and the pulp are potentially recovered in the mechanical depulpment in a percentage of 43.2%, the mucilage in the demucilaging stage in 11.8%, and the parchment after the drying and dehusking in 6.1%.19 The silverskin is only removed after the coffee roasting process. This operation is considered extra to the wet processing stages carried out in Honduras.16 The adapted Table 1 of Iriondo-De-Hond et al.19 summarizes the number of by-products generated in the wet and dry processing and the bioactive compounds and nutritional compositions present in each one.
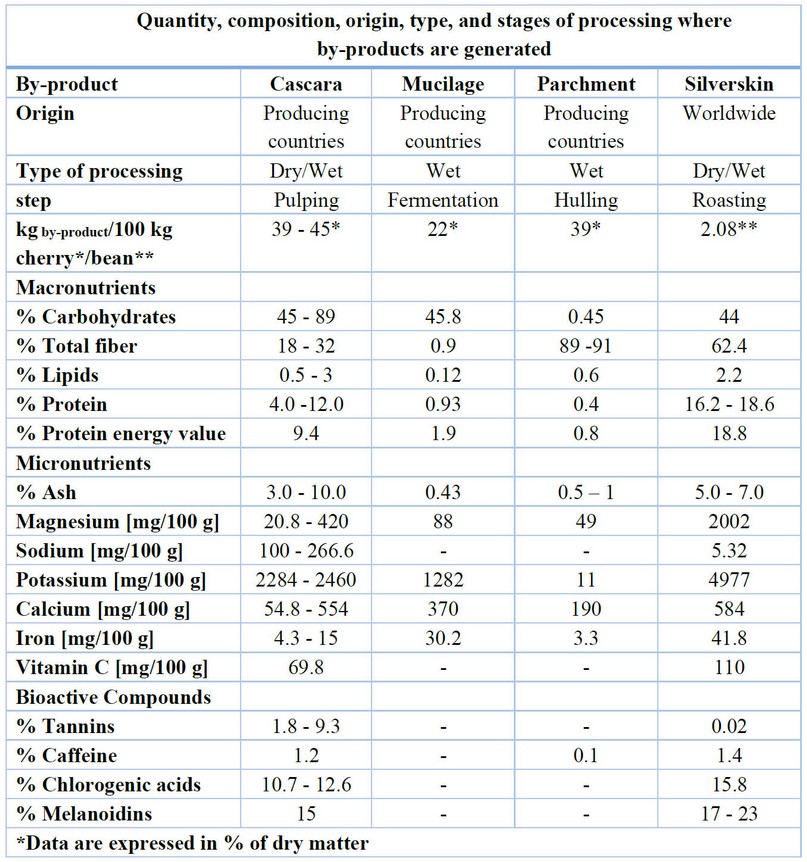
Table 1. Nutritional composition and bioactive compounds of coffee by-products. The date is expressed in dry mass percentage.
The environmental and social impact of coffee processing, added to the increase in its production, has aroused interest in organizations, governments, producers and society to pay attention to by-products. To save resources in the agri-food sector, the Food and Agriculture Organization (FAO) suggests implementing a waste hierarchy that reduces the by-products. The most recommended actions are prevention, reduction, reuse, and recycling. The least recommended action is incineration and landfills.20
Coffee can be used in ethanol production via traditional fermentation through yeasts from two substrates: fresh and dry husks. A total amount of 14 and 29% in sugars on a dry basis for these substrates has been reported, respectively. In 2009, Gouvea et al. conducted fermentation studies, concluding that the mentioned substrates are a potential source for ethanol production compared to other residues. They recommend analyzing these wastes for potential substrates destined for biorefinery.21
In the same year, Murthy et al. reported the solid-state fermentation (SSF) of the husk, pressed pulp, parchment, and silver skin as substrates and the Neurospora crassa as the fungal strain to obtain the α-amylase enzymes that show acceptable enzymatic activity.22 Additionally, the same research group reported the extraction of phenolic antioxidants from these coffee wastes employing mixtures of solvents and pretreating the wastes with mixtures of cellulolytic enzymes (lysozyme), obtaining mainly chlorogenic acids (CGA). The silverskin reported the most significant yield in the extraction, revealing that these by-products can be a source of extraction of other bioactive compounds of interest, such as nutraceuticals and food preservatives.23
Other authors have found other applications of industrial interest for the coffee by-products. Among the many applications found, some of them are food coloring,24,25 obtaining honey and coffee flour,26 as a fiber source in biscuits and bakery preparations, and 27,28 as potassium and vitamin C sources through the manufacture of instant drinks.29
Oliveira et al. used dry coffee husks as a heavy metal bio-adsorbent in the materials field. The heavy metal ions in the aqueous solution were copper, zinc and chromium. They report a successful sorption for low concentrations of the ions.30
The coffee husks have been used as biomass for energy production. For example, the husk with the least mucilage reported a high calorific value of 16.1MJ/kg. On the other hand, parchment reports a high calorific value of 18.2MJ/kg. However, these results may be affected by its low bulk density, increasing storage and transportation costs.31
African oil palm
African oil palm, or macaw-fat or just oil palm, is a tropical plant that grows in lands with altitudes less than 500 meters above sea level. Its origin can be traced back to the Gulf of Guinea in West Africa, hence its scientific name, Elaeis guineensis. Its introduction to the American continent is attributed to the Portuguese colonizers and traders due to the presence of oil palm in their diet.32
Palm oil is Honduras’s second most exported agro-industrial product; in 2021, a total export of 490.81 million kilograms was reported, equivalent to US $554.4 million in the Global Market.1 Honduras is one of the leading palm oil producers, ranking eighth in world production and third in all American countries, bested only by Guatemala and Colombia.
African oil palm cultivation in Honduras began in 1929 when the United Brands Company started planting seeds of several strains of Malaysian origin. These seeds were planted at Lancetilla Botanical Garden in Tela, Atlántida Department. The first oil palm plantations with for-profit purposes were established in 1936 and 1938 in El Progreso, Yoro Department. In the ’70s, the oil palm industry began to rise with a project of 11,000 hectares in the Aguan Valley in Colon Department, and in the ’90s, growth in industrial development was prompted due to companies and independent producers uniting to create Federación Nacional de Productores de Palma de Honduras (FENAPALMAH).32
Until 2016, it was estimated that 189,500 hectares were planted, 155,000 were in production, and the rest were still in development. The main product of interest of the oil palm is the crude oil contained in its fruit. The fruit has an ovoidal shape, is 3 to 6 cm long, and weighs about 5 to 12 grams; it has red-colored skin, and its diameter can go up to 4 cm. It presents a smooth and shiny epicarp, a mesocarp or fibrous tissue that contains lipids within their cells, an endocarp or nut comprised of a lignified shell and an endosperm or oily almond, as seen in Figure 4.
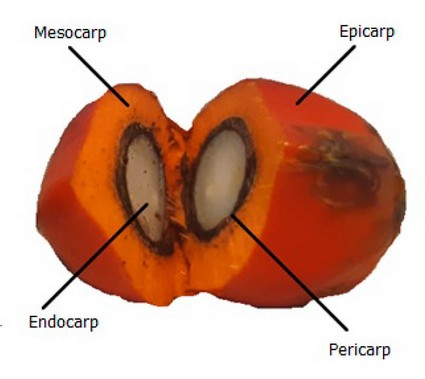
Figure 4. Vegetal morphology of the fruit of Elaeis guineensis variety tenera.
The African oil palm is a perennial plant with three varieties: dura, pisifera, and genera. The general variety is used in crude oil extraction and comes from a cross between two varieties, dura and pisifera; its general characteristics are shown in Table 2.32 The dura variety has a mesocarp that takes about 35-50% and reaches 65% of the fruit. Nevertheless, the mesocarp’s oil content is low concerning the fruit, making only about 17-18% of its weight. The psifera variety can be distinguished because it has a fruit without an endocarp and has fibers surrounding the endosperm. The tenera variety has an endocarp with a thickness of 0.5 to 4 mm, and the mesocarp has a high weight proportion of 60-96%, but the proportion of crude oil per bunch, usually between 22-25%, with the best plants reaching up to 30% of the whole bunch weight.33
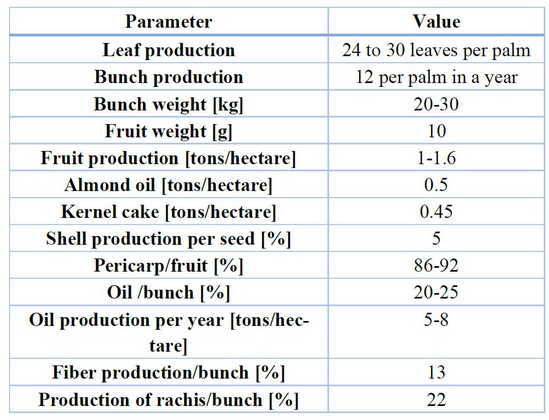
Table 2. General data of the African oil palm Elaeis guineensis variety tenera.
In the cultivation of the African oil palm, there is a germination period between 75 to 90 days, and then they are transferred to a nursery where they remain from 10 to 14 months. The adequate initial development in this phase directly influences the behavior of the seedlings in the field and will affect the production of bunches during the first years of harvest. The harvest is carried out throughout the year as long as it is considered that the fruit has matured enough since this guarantees a higher content of lipids. The change from a purple to a reddish-orange color in the fruit signifies correct ripening. The harvest can take between 30 and 36 months from when the palm is planted in the field. The harvesting cycles can vary depending on the age of the palm and the regional climatic conditions; these are more frequent in rainy seasons.34 A palm is considered young when it is under five years old; it produces 14 bunches per year, weighing 7 kg per bunch, increasing to about 22 kilograms when the plant reaches eight.35
After harvesting, the fruit is processed at an extracting plant to obtain the crude palm oil; in some plants, the crude oil is refined to obtain refined, bleached, and deodorized oil (RBD oil). At the extracting plant, the first stage is the reception of the bunches, where most of the impurities coming from the field are removed. The bunch goes through a machine that fractures it into several pieces, allowing the fruit to fall out and separate from the rachis, which is the fibrous structure that supports the fruits. The crushed fruits and rachis are sterilized to maintain the crude oil quality and detach the seed from the fruit. Presses extract the crude oil from the sterilized fruit, obtaining solid organic matter as a by-product. The crude oil is dragged by hot water to maintain its temperature and goes through a clarification process.36 After the extraction process, the crude palm oil is ready to be refined and transformed according to the objectives of the production plant.
The crude oil contains a balanced composition of saturated and unsaturated fatty acids with 4% monounsaturated oleic acid, 10% polyunsaturated linoleic acid, 40% saturated palmitic acid, and 5% saturated stearic acid. It contains antioxidants in the form of vitamin E and vitamin A.37 The solid mass is kernel cake, separated into rachis fibers and seeds. The seeds are taken to the palmistry section, where they are broken to separate the kernel from the shell to obtain palm kernel oil. The fiber of the rachis, the shell, and all the seed fibers that have gone through the oil extraction process are dried and used as fuel for boilers.38
Crude palm oil is one of the oils with the best relationship between production cost and yield per hectare.39 Until 2016, fifteen extraction plants were known in Honduras. Six plants produce RBD oil, and five offer manufactured products to be marketed, as shown in Table 3. These oil extraction plants showed a yield of approximately 20% in terms of oil recovery, which means about 520,000 tons of oil come from an annual production of 2,550,000 tons of palm, for which a yield of 16.45 tons of fruit was estimated fresh per hectare.40
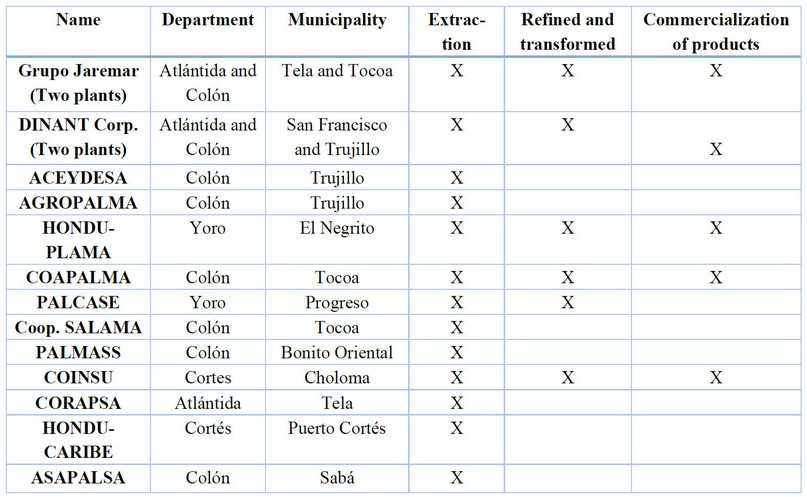
Table 3. African palm oil extraction in Honduras.
According to the National Federation of Oil Palm Growers (Fedepalma) in Colombia, four general sectors of palm biomass utilization can be identified: energy (biofuel and electricity), manufacturing (also known as eco-products), agriculture (animal feeding and compost), and biotechnology (high-value chemicals and activated carbon).41
Due to the vast quantity of palm planted on the Honduran soil and the high yield of oil extraction, a significant amount of biomass is produced, mainly utilized as fuel material for the boilers of cogeneration systems in the oil extraction plants. However, this is only a measure to reduce the amount of waste and cannot utilize all the biomass potential as renewable energy. Table 4 shows the physical and chemical data of the main components of the biomass used in extracting plants.42 The oil that remains in the seed and cannot be removed is also considered.43
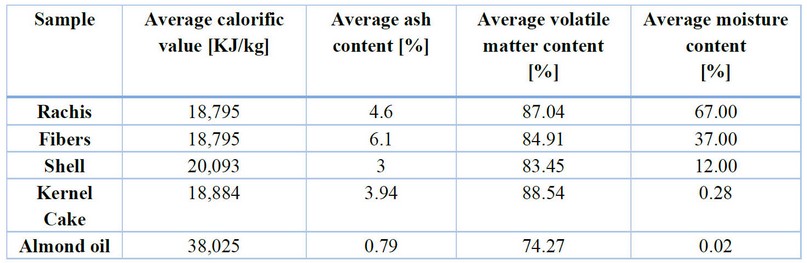
Table 4. Energy data of biomass from African oil palm.
The high energy content of the oil is due to the amount of long-chain fatty acids of about 16-18 carbon atoms. However, many of the significant African palm oil producers around the world, such as Malaysia, Indonesia, Thailand, Colombia, and Ecuador, promote the use of the oil residues contained in the biomass for biodiesel production because the seed has a significant amount of short-chain fatty acids (8-10 carbon atoms) and medium-chained fatty acids (12-14 carbon atoms). This biodiesel presents a comparable quality to the biodiesel produced from rapeseed oil. The latter oil is primarily utilized for biodiesel production.44
Biogas is another possibility of taking advantage of the liquid biomass stored in the effluents of extraction plants and oxidation ponds or biodigesters for energy generation.41 The transformation of biomass to gas (BTG) has tested and accessible technology for its direct use in boilers, power generators, and other equipment. In Latin America, Honduras and other Central American countries are leaders in energy development and have found a viable option for economic growth.
Another significant residue from the extraction process is the rachis, a part of the harvested bunch. In 2017, the rachis was evaluated as a material that can adsorb certain dyes found in wastewater. The effectiveness of rachis adsorption was studied with and without chemical pretreatment by controlling pH conditions, dye concentration, and the ratio between the rachis and the dye in the wastewater. This study concluded that the palm rachis is an effective adsorbent of textile dyes, reaching a dye removal between 60 and 85%.45
Other more specific uses that can be given to African oil palm residues are those related to nanotechnology. One of these technologies is the manufacture of activated carbon. Furthermore, the presence of essential molecules in palm oil matrices makes them good candidates as stabilizers for nanoparticle formation. Crude and fruit oils have facilitated the biosynthesis of silver, zinc oxide, and gold nanoparticles, which have applications as reducing agents, stabilizers, and adsorbents. Carbon-based nanomaterials can also be generated due to the chemical composition of African palm biomass, as shown in Table 5.
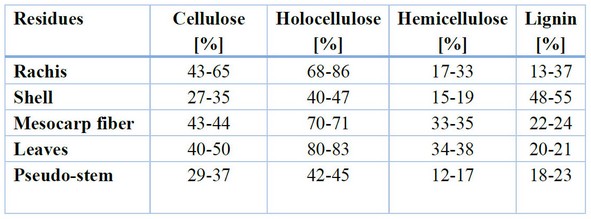
Table 5. Carbohydrate distribution in African palm residues.
In the Biotechnology field, the cellulose extracted from this biomass manifests a high resistance and a low density, which are attractive physical and chemical properties and present the advantage of having a low cost and being biodegradable. Some materials it can synthesize are nano-cellulose, nanocrystals of cellulose, carbon nanotubes, graphene, and activated carbon. These materials have a wide range of uses, such as supercapacitors, complements in biocomponents, production of polypropylene compounds, concrete reinforcement, and treatment of effluents of oil extracting plants.46
Biomass in other applications is reported, including paper manufacturing and its use in specialized products such as mattresses, filling materials, the automotive industry, and plastics. In agricultural applications, biomass can be used as a composting method. In animal feeding, some new combinations of kernel cake mixed with vegetal material from the leaves are used to produce animal feed pellets.
Sugarcane
Sugarcane is a plant that significantly influences Latin American countries through seeding, harvest, and transformation.47 The sugarcane is a tropical grass of high altitude, commonly related to sorgo and corn. A juice rich in sucrose is accumulated in the plant, and this is transformed within the sugar mills. Sugar is the resultant product of the harvest of sugarcane leaves, which has an approximate growth between six and eight months, depending on the sugarcane variety. In Honduras, the variety grown of sugarcane is S. officinarum. The main section on which the transformation and production of sugarcane is focused is the stem, which is divided into nodes and internodes, as shown in Figure 5.48,49. The internodes comprise the solid part known as fiber and the liquid known as juice, which contains water and sucrose, as well as other components in small amounts, as described in Table 6.
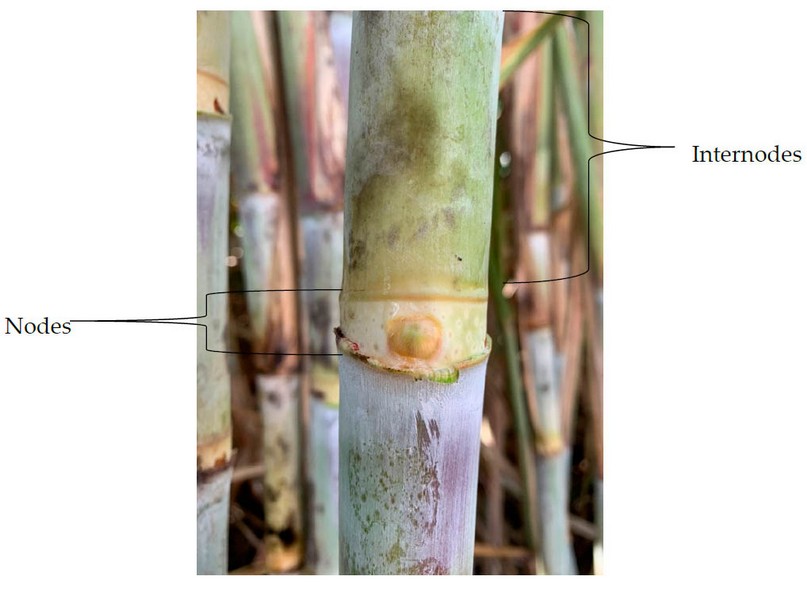
Figure 5. Nodes and internodes of sugarcane stem.
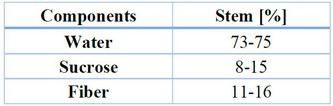
Table 6. Main components of the sugarcane stem.
The sugarcane in Honduras is harvested manually by burning and optimized using mechanized machines. By having an adequate high, the sugarcane is transported to the sugar mills for transformation, combined with the leaves, corolla, and branches, which can affect the transformation process in the mills. In Honduras, the sugar mills, with the passing of the years, have been adapting their technologies, trying to reduce the waste and by-products generated in the production process. The principal product of the transformation of the sugarcane is sugar, which is distributed in the local and international markets for later use in other processes.48 During the transformation, some by-products are generated, the most important being molasses due to its high commercial interest. Other by-products are also generated, such as bagasse, bacilli, and cachaca, as shown in Figure 6. These by-products were studied in the last few years to find new alternatives to the green process and reutilization. To a lesser extent, the bagasse and the bacilli continue to be used in mills as the primary source of combustion for steam generation in boilers.50 Instead, the cachaza, the by-product generated in the separation process of clarification and filtration with a high content of humidity, around 70 g per 100 g of filter cake, has found alternatives as fertilizer for the farmlands and as a supplement for the feeding of ruminant’s animals.51 In Colombia, a study was conducted to use the cachaza as a nutrient material for the elaboration of compressed earth by using the precipitation method, a process which can generate precipitates of calcium carbonate and as a component for bioremediation process in fields with high content of aromatics hydrocarbons and total hydrocarbons.52
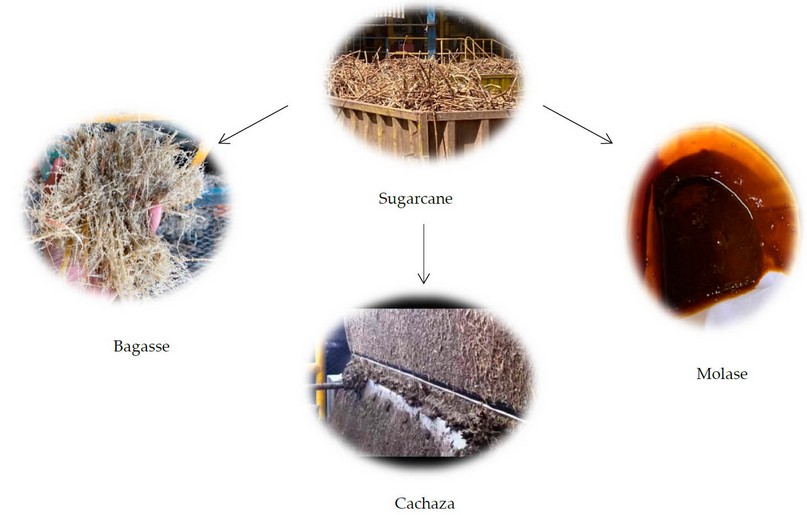
Figure 6. Sugarcane by-products.
In Honduras, according to the Secretary Department in the Energy Office report, the current generation of renewable energy is based on 7.78% produced by biomass, a category to which the bagasse belongs, in comparison with hydraulic energy (26.31%), wind energy (8.39%), photovoltaic energy (19.04%) and geothermic energy (1.30%)53. According to the Balances Energéticos Nacionales (BEN), in 2017, it was estimated that 2.98 million metric tons of bagasse were used to produce energy, representing an equivalent of 3096 barrels of petroleum (BOE).54 In Honduras, solar, firewood, wind, hydroelectric, and bagasse are primary energies. The latter represented 23% in 2017. Of this percentage, 48% was used by the industrial sector and the rest was distributed by the Empresa de Energía Honduras (EEH). During 2017-2018, bagasse reached 30% within the primary energies.55 In 2019, this percentage decreased to 11%. In 2020 because of the pandemic of COVID-19, reported a reduction of 9%, which was all consumed by the local industry.56,57
The sugar mills located in Choluteca, Francisco Morazán, Cortés, and Santa Bárbara study the biomass of the surroundings for the combustion in the boilers, focusing on properties like caloric power as a principal physiochemical parameter for58 The bagasse contains the most quantity of fiber inside the sugarcane after partially or entirely removing the sugar juice. The caloric power depends on the physicochemical composition, the percentage of humidity, and the residual components as volatile compounds and ashes, in addition to the particle size before the combustion in the boilers. The dry bagasse was estimated to have a gross calorific value (GCV) of 19,605 kJ/kg.59 Inside of the common parameters in the sugar mills, it was estimated that the humidity can be in the range of 47-56%, affecting the GCV, reporting values between 7500-8400 kJ/kg for the wet bagasse.60–62 The caloric power can be improved through the methods of gasification and pyrolysis, which are focused on removing water.63 In Mexico, a study was conducted using the biotransformation with carbon to increase the caloric power of the solid mixture, obtaining better results than the bagasse as biomass.64 Table 7 reports a typical physicochemical and elemental analysis of the bagasse.48
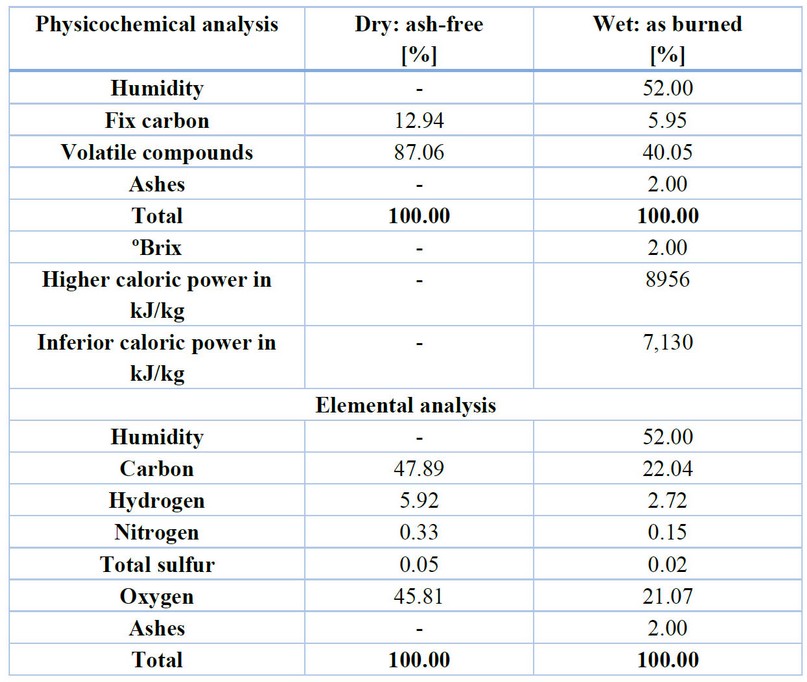
Table 7. Adaptation of typical physicochemical and elemental analysis of bagasse.
Barroso et al.58 estimated that 280 kilograms of bagasse waste are generated from one ton of sugarcane. The leading destination of this bagasse is waste or energy generation by biomass combustion.
It is well known that the cement industry causes 5% of the carbon dioxide that coexists in the atmosphere. Thus, various investigations in the field, like Asma et al.,65, have been trying to find new alternatives for the materials used in the production process to reduce the gas emission percentages. The same study has shown that the chemical composition of bagasse, after thermal transformation by pyrolysis, contains pozzolanic materials, which are helpful in cement fabrication. The sugarcane bagasse ash (SCBA) has also been studied in India to make cement with different proportions of bagasse ashes, which has shown satisfactory results in increasing the mechanical properties and in the energetic savings in the production process. In Honduras, as Bonilla and Borrachero68 indicate, a study was made with ashes samples coming from Santa Matilde Sugar Mill and Los Mangos Sugar Mill in Choluteca, finding elements like quartz, albite, magnetite, mullite, and cristobalite in the composition of the ashes. This result opens a potential opportunity for the Honduran companies to improve the investigation in cement production.68
Another alternative for the bagasse is its introduction as a raw material to produce second-generation bioethanol (2G) in Brazil.69 According to studies at laboratory scale, the lignocellulose biomass has the potential to produce up to 50% more ethanol compared to the first generation of bioethanol, which is produced from sugarcane juice and corn juice fermentation. However, technological difficulties and scalability problems have been encountered in using the bagasse biomass to produce bioethanol at the industrial level because of the high energy cost and the use of hydrolase enzymes to decompose the matrix of the bagasse in its fundamental components.70,71 It has been encountered that the bagasse can be used as a medium culture for insects, yeast, and mushrooms and as a substrate for industrial enzymes, paper pulp, and the production of other essential commodities.72 As a complement for ruminant food51 and for the value of lignin that can be used for medical purposes.73,74 In Honduras, there are no studies for the development of bagasse for medical purposes, but there is a study that evaluates the use of bagasse to produce biogas75. According to this study, there is a production potential of 19.3 Nm3 (standard cubic meter of gas) of biogas to 60% in methane per year for the production in the harvest of 2009-2010, considering the effluents, the water that is treated in the production plants and waste of energy that goes in the bagasse and cachaza in solution. This amount equals 26.4 MWt (thermal megawatts) as an available potential for thermal energy in six months per year. However, this study shows a more excellent production of energy if the molasses is used as a source of biogas, around 75.8% of methane. The difficulty is that the molasses is used as a raw material for other transformation processes, and its sale is more profitable than its production as biogas. Table 8 describes the energy potential of biogas based on the study described above of the sugar mills in Honduras.
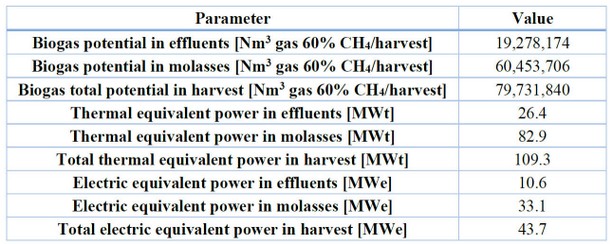
Table 8. Adaptation of study of biogas in Honduras.
Due to its variety of essential chemical structures, bagasse can be used as a biopolymer substrate for generating melanins with the help of melanogenic marine bacteria. Studies of the tequila agave bagasse showed that the bagasse can produce polyhydroxyalkanoates (PHAs) when consumed as food by microorganisms responsible for the production of the biopolymer or as a candidate for obtaining phenolic compounds such as vanillin, 3,4- dihydroxyphenylalanine (L-DOPA), gallic acid and salicylic acid.76
Due to the value-added components within the bagasse, various transformation studies have been developed to extract these components and use them as raw materials for other industries.48 It is remarkable that the transformation cost is elevated in some processes. However, they open the window for utilizing the by-product not only for energetic purposes but also as an alternative material for production.
Shrimp
Shrimp is a seafood rich in protein and is widely consumed around the planet. According to the 2020 Food and Agriculture Organization (FAO) report, shrimp is among the four most commercially valued crustaceans. Asia remains the largest producer of aquatic animals, including shrimp, with 80% of its world production, followed by America.77,78 In Central America, Honduras leads aquaculture production, followed by Nicaragua.79
Shrimp production in Honduras began in 1969 with the Armour United Fruit Company on the country’s north coast. In 1972, the company Sea Farms moved the production to the country’s south, specifically to the Gulf of Fonseca. Honduras has a shrimp industry of approximately 420 projects cultivated in an extension of 24,500 hectares of land.80
Shrimp ranks third among agro-industrial products with the most significant exports, after coffee and palm oil. In 2021, the Asociación Nacional de Acuicultores de Honduras (ANDAH) reported exports of over 59 million pounds of shrimp, with the European Union and Taiwan as the main destinations.81 In December of that same year, the Banco Central de Honduras (BCH) reported that US$267.2 million was collected from shrimp exports.1
The shrimp species produced in Honduras is Litopenaeus vannamei, known as pacific white shrimp or whiteleg shrimp. This species is found along the American coast of the Pacific Ocean from the Gulf of California to the Equator.82 The white shrimp life cycle has the following stages: a larval stage, a postlarvae stage that evolves into a juvenile, and an adult stage.83 Shrimp production in Honduras is based on two mechanisms: the collection of wild postlarvae and the production of postlarvae in laboratories. The latter provides the highest percentage of shrimp production in Honduras. Regardless of their source, postlarvae are stocked directly into a production pond. The culture is classified as extensive, semi-intensive and intensive depending on the number of postlarvae per square meter or crop density. A low density of postlarvae characterizes extensive cultivation. On the other hand, intensive culture has a high density. In Honduras, two types of cultivation are developed: the extensive one in the artisanal sector, with a contribution of 20% in production, formed mainly by small and medium-sized producers, and the semi-intensive one, with a contribution of 80%, formed by large-scale producers. Shrimp production extends throughout the year but peaks between May and October. Each cycle lasts approximately 12 weeks, varying depending on the production type carried out in the pond.84
The external anatomy of the shrimp can be divided into three main parts: the head, the thorax, and the abdomen. The head, together with the thorax, form the cephalothorax, and the entire body of the shrimp is covered by an exoskeleton, as shown in Figure 7.85
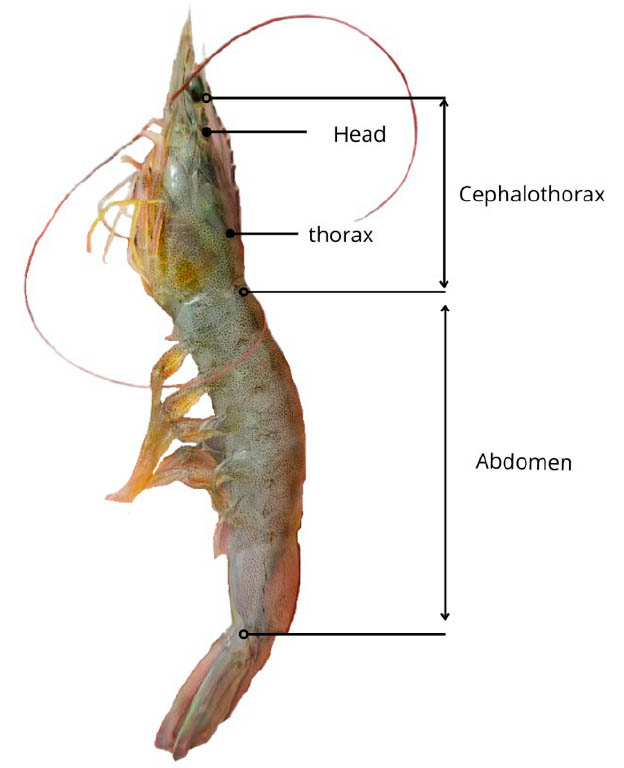
Figure 7. The main structure of Litopenaeus vannamei shrimp.
Honduras currently has 13 shrimp packing plants, all located in the southern part of the country.86 In these processing plants, it is considered on average that 35% of the total mass of the shrimp corresponds to the cephalothorax, known locally as the head, and 15% by mass to the exoskeleton, known locally as the shell and tail, the rest of the percentage by mass corresponds to the abdomen.
Shrimp in Honduras is exported in different presentations: whole, headless, peeled, and peeled-precooked. The choice of product type depends on world demand. The highest percentage of exports occurs for whole shrimp. However, headless and peeled shrimp has increased in recent years, as shown in Figure 8.87
The cephalothorax and the exoskeleton resulting from the different presentations’ production processes are considered waste. On average, between 2016-2021, there was a production of 101 million pounds of shrimp; 35 million pounds are considered waste, equivalent to 34% by mass, as shown in Figure 9.88 In general, the composition of shrimp waste depends on the species and the geographic region.
Duan et al. 89 classify Litopenaeus vannamei shrimp’s waste composition in Table 9, showing that proteins, minerals and Chitin are the main components.89 This same composition on a dry basis corresponds to 49% protein, 23% minerals, 7% lipids and 20% chitin.
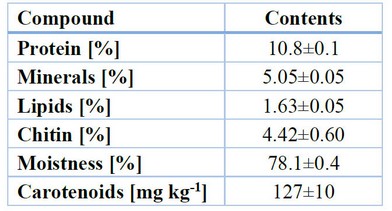
Table 9. Waste composition of Litopenaeus vannamei shrimp.
Currently, the shrimp waste generated in the packing plants of Honduras is received in the Procesadora Hondureña de Alimentos de Camarón (PROHALCA SA). Due to its high protein content, this waste is used and transformed into protein for animal consumption. However, its chemical composition shows that these wastes can also be given other applications. Next, the potential uses are described in materials science and energy for the waste components in Table 9.
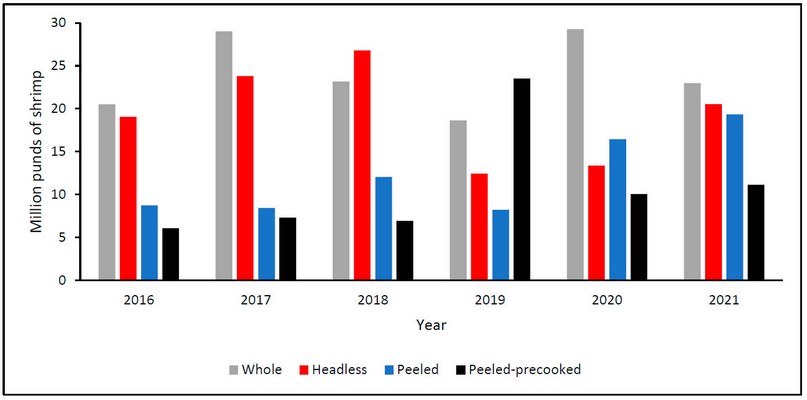
Figure 8. Exports in Honduras of shrimp in different presentations in millions of pounds: whole (gray), headless (red), peeled (blue), and peeled-precooked (black) from 2016 to 2021.
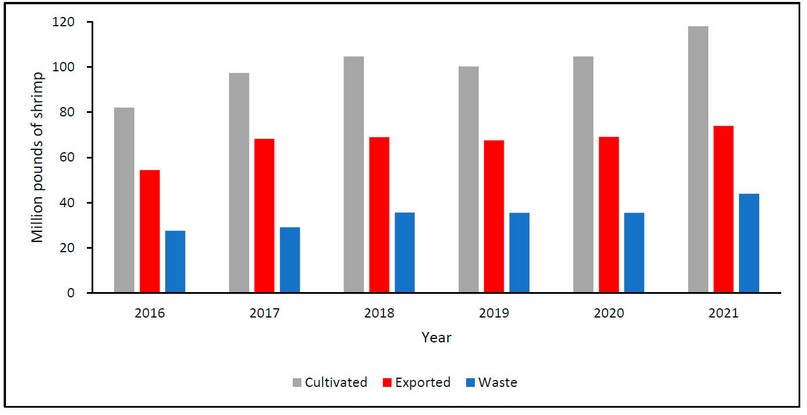
Figure 9. Shrimp production in millions of pounds in Honduras: cultivated (gray), exported shrimp (red), waste (blue) from 2016 to 2021.
Chitin is the second most abundant biopolymer in nature after cellulose. On the other hand, chitosan is a chitin derivative produced upon deacetylation of the compound. 90,91 Chitin can be used as the only carbon source for the production of biofuels, and its derivatives are used in the biomaterials industry to manufacture bioplastics.92 Chitin has applications in the food industry in packaging that have antimicrobial properties93, in the textile industry,93 in the agro-industrial sector, improving crop yields thanks to its pesticide effect95, in water and wastewater treatment,96 and in biomedical applications for its biocompatibility, biodegradability and non-toxicity. Some investigations report the use of Chitin as a nanomaterial for drug transport and cancer diagnosis.93,94
Proteins are the most abundant components of shrimp waste. For the species Litopenaeus vannamei, Gómez-Estaca et al. report the recovery of approximately one-third of the essential amino acids. The amino acids found with greater abundance are glycine, glutamate, aspartate, and alanine.95 This combination of amino acids is comparable to soy-based meals, making shrimp waste an attractive option for production for feed additives for aquatic animals, farm animals, and poultry.96
Calcium is the most abundant element found in the shrimp waste of the species Litopenaeus vannamei. However, phosphorous, sodium, potassium and magnesium amounts are also reported. Heavy metals such as zinc, iron, copper and manganese are found in lower concentrations without health risk.95
The cephalothorax is the lipid source of the shrimp. Fatty acid profile analysis shows that the most abundant polyunsaturated acids present are oleic acid, linoleic acid, and palmitic acid, and the levels of docosahexaenoic acid (DHA) and eicosapentaenoic acid (EPA) were also notable.97
Carotenoids are responsible for the coloration of both the external and internal surface of the shell of crustaceans.98 Astaxanthin is a reddish-pink pigment from the group of xanthophyll carotenoids found in shrimp.102 Astaxanthin is fat-soluble; it is present in the lipid extract of shrimp cephalothorax.100 This pigment is a vitamin A precursor99 and a powerful antioxidant.100 The antioxidant properties cover several aspects of human health, such as protection against ultraviolet light and anti-inflammatory. In addition, it has essential applications in the nutraceutical, cosmetic, food, and animal nutrition industries.101
The waste generated in the commercializing sea products, such as shrimp, has been used in alternative sustainable technologies for energy generation. An example is the study of Kannan et al., which used microwave hydrothermal carbonization to convert moisture biomass waste from seafood into a carbon-rich product called hydrochar. Microwave hydrothermal carbonization is a modification of hydrothermal carbonization. The latter is characterized by working under humid conditions, temperatures between 150 °C and 250 °C and high pressures; it has the disadvantage that the process times are long. On the other hand, microwave hydrothermal carbonization takes advantage of the moisture content to heat the biomass from the inside out with shorter exposure times under the principle of dielectric heating.102
Cashew
The cashew, with the scientific name Anacardium occidentale, is a native fruit to the Northeast of Brazil; it is known worldwide for its fruit and seed, which has led to its broad commercialization, being mainly produced in all tropical areas, of which the countries of India, Brazil, Mozambique, Kenya, Tanzania, Australia, and Vietnam have the most significant extension of cultivated area in the world.103 The cashew nut is among the nuts with the highest production worldwide; 104, its export is mainly destined to North America, Europe, and some Asian countries.105 In developing countries, its production is promoted, representing a socio-economic benefit.106 It is also a dried fruit in high demand due to various factors, such as its implementation in healthy habits due to its folic acid, unsaturated fats, fiber, proteins, potassium, phosphorus, and magnesium content.105,107,108 It is also considered a multipurpose product since it presents possible applications in multiple industries such as medical and pharmaceutical industry, food industry, and agricultural industry, to name a few.109
Honduras has a favorable territory for agricultural exploitation. The departments of Valle and Choluteca, located in the region of the Gulf of Fonseca, in the southern part of Honduran territory, are the most important for cashew production, with an approximate number of 1,163 producers. Based on a study in the Gulf of Fonseca in 2013, approximately 13,000 quintals of cashew seed per harvest are produced.110 The countries that import the cashew nut produced in Honduras are Guatemala, Nicaragua, El Salvador, Germany, and the United States of America.111
Among the varieties of cashew cultivation, red and yellow fruits are known, the latter being less astringent than the red ones. Similarly, varieties according to size may be classified. 111 Cashew comprises a fruit and a nut; both components have been used in different industries, such as food, pharmaceuticals, and cosmetics.112,113
The cashew fruit, known as the false fruit or pseudocarp, is the majority of the cashew; it’s pear-shaped and has a bright yellow to red color when ripe. Ripe cashew fruits are fleshy and have a sweet to bitter, astringent taste and a strong aroma. The cashew shell is grayish with the shape of a kidney when it reaches its maturation, which occurs before the maturation of the fruit. It contains the cashew nut inside, as shown in Figure 10,111, being the most consumed part and considered the main product of the crop.103
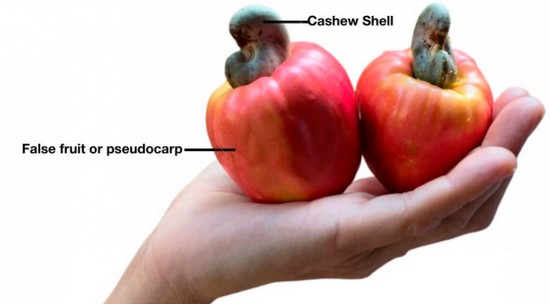
Figure 10. Plant morphology of cashew
In the process of obtaining the cashew nut, the nut is separated from the cashew shell, the latter being generally discarded as waste, causing an environmental problem. Some applications that can be given to the cashew shell are the generation of energy and the elaboration of new products since it is also a rich source of compounds of interest in the chemical and pharmaceutical industry,108 offering great medicinal and commercial value.111
The raw nut contains acids that could cause burns in the mouth, so it goes through a roasting process before consumption, causing variation in its antioxidant activity and phenolic extract content depending on the roasting temperature.109 In addition to its pleasant flavor, the cashew nut is widely consumed due to its nutritional properties, properties that are related to the high content of lipids that have been shown to contribute to the reduction of LDL (low-density lipoprotein) cholesterol, presenting relevant health benefits such as reducing the risk of developing cardiovascular diseases,114 preventing some types of cancer,115 nervous system protection,115 antioxidant action,116 and source of vitamin C.113,117
Due to its nutritional properties, it has taken protagonism in the diet of consumers interested in improving the quality of their diet109 and is considered a source of high-quality protein. It is rich in polyunsaturated fatty acids, fats and carbohydrates and has a high energy value and high levels of minerals.116 It should be noted that it has been found that the nutritional value of the cashew nut differs according to its origin and the roasting process.109 Rico et al.104 determined the nutritional value of cashew nuts from Brazil, India, Ivory Coast, and Vietnam, finding that, in general terms, the main component of the nuts studied were total fats, most of these being unsaturated. The second most abundant component was proteins, followed by carbohydrates. Amino acids were also found, being the one with the greatest presence, glutamic acid. Among the vitamins, the most abundant was E, and among the minerals found, potassium was the most present, followed by phosphorus and magnesium.104 Other studies coincide with the nutritional composition mentioned above.118,119,120 As a result of several studies, it has been found that the average energy content of the cashew nut is greater than 2000 kJ/100g.119,109,121
The false fruit represents 90% of the total weight of the cashew. It is mainly consumed as a direct fruit or as a fresh drink due to its high vitamin C.113 It is processed for desserts such as dehydrated fruits, jelly, gelatin, sweets, honey or vinegar and wine production.111,112,113 In many developed countries, it is also used to produce ice cream, vegan meat or the base of soups and creams.110 The composition of the false fruit is very complex and is characterized by containing a high percentage of ascorbic acid (vitamin C); it is even estimated that it is five times richer in vitamin C than citrus fruits and four times richer than sweet orange. In addition, it contains considerable levels of minerals, mainly calcium and phosphorus.118 It also contains tannins, organic acids, carbohydrates, and polyphenolic compounds known for their antioxidant health benefits,113 both for human consumption and for the production of meals for animal feed.109 Due to its content of proteins, fatty acids, crude fiber, pectins, and flavonoids, it has been reported that by-products resulting from the processing of cashew nuts and their juice, such as bagasse (20% of the fruit), have been used in the production of bioethanol, biodiesel, dyes, pesticides, larvicides, repellents, and adhesive resins.109
When the cashew nut is extracted, the shell that remains as residue has an extractable liquid that contains many components that can be used due to their high-value chemical structures. The cashew nut shell liquid (CNSL) represents approximately 25% of the total weight of the cashew nut.122 It is considered one of the few primary and economic sources of natural phenols that constitute a promising alternative to petroleum-derived phenols. CNSL comprises anacardic acid, small amounts of cardanol, and methylcardol.122 These compounds have three reactive sites: a phenolic hydroxyl group, an aromatic ring and a long aliphatic chain in the meta position, which can be saturated, monounsaturated, unsaturated, or tri-unsaturated. The structural characteristics of these compounds are of great interest since they can replace phenol in many applications with similar or better results.122
Anacardic acid has aroused the interest of researchers due to its nature as a phenolic compound presenting antioxidant activity.123 it also has high pharmacological values such as antitumor activity113, antifungal activity113 and anti-inflammatory.109 Its structure can be considered a structure derived from salicylic acid substituted by a long chain of hydrocarbon, providing a more excellent lipophilic character conferring interesting properties, presenting a high antibacterial, antimicrobial,124 gastro-protective, anticancer, larvicidal,125 anti-inflammatory,109,125 inhibition of histone acetyltransferase activity.109 Studies have shown that the mixture of carotenoids and anacardic acids can be beneficial as an anti-inflammatory and antiulcerogenic.126 On the other hand, studies have been carried out on the potential use of anacardic acids for the treatment of dental caries and acne, as well as in anti-cancer treatments and control of tiny pests in crops.127 Given the benefits it has been shown to have, anacardic acid can be a starting point for developing local drugs in Honduras, promoting the country’s pharmaceutical industry using and taking advantage of the cashew shell, which is now considered a residual waste.
Cardanol represents a lower percentage by weight of CNSL compared to anacardic acid and cardols.122 However, a decarboxylation reaction can transform anacardic acid into cardanol. The latter is the component that presents the most extraordinary versatility for its subsequent transformation, cataloged as a «building block,» which, with various chemical transformations, can be converted into a large number of compounds that have several applications, such as surfactants, antioxidants, fuel additives, liquid crystals for optical materials, fibers, among others.108,122,128 In addition, the transformation of cardanol to essential intermediates in the processes of resin synthesis, polyurethane synthesis, latex synthesis, as a drug precursor, co-surfactants and surfactants, as a plasticizer for the synthesis of soft polyvinyl chloride (PVC), as an additive for cellulose diacetate, flexibilities, fuel additives, among others, have been reported.128,109
It is possible to notice the versatility of cardanol and its derivatives through its ability to participate in a wide number of chemical processes and transformations, strengthening interest in the industrial sector by presenting the advantage of being a natural and renewable compound, specifically in a country like Honduras without explored oil reserves, taking advantage of these natural phenolic compounds. On the other hand, cardol, present in small quantities in the CNSL, has presented applications in the plastics, insecticides, and inks industry.129
Biodiesel obtained from the CNSL in the cashew nut does not require transesterification or further processing. In addition, studies have been carried out on implementing biodiesel from the CNSL mixed with ethanol, resulting in a significant reduction in gas emissions: 27% CO2, 57% NO and 8% hydrocarbon conferring this characteristic to the cooling effect of ethanol.109
The renewable energy research field has boomed in recent years, intending to increase energy requirements and environmental regulations. The cashew shell has a calorific value greater than 23.43 kJ/g before extracting the CNSL. Once the CNSL has been extracted, the calorific value adopts ranges higher than 4.10 kJ/g, which is still usable for energy production.121
On the other hand, the cashew’s tree trunk can also be used since it has a high content of lignin (25%) and hemicellulose (35%), which is reflected in an approximate calorific value of 20.42 kJ/g130, allowing its potential use as firewood in many Honduran homes, as well as a possible power source for industrial equipment.
Another alternative for implementing the cashew as an energy source is using the false fruit and the cashew shell in gasification processes. After a qualitative evaluation of several implemented residual wastes, a study by Chávez et al. reports that the cashew shell represents the most excellent viability for the gasification process because it exists in large quantities and is not currently used in other industrial processes. In turn, it is of simple harvest and previous treatments.121
CONCLUSIONS
Using biomasses derived from agro-industrial residues for producing energy and chemical products is presented as a possibility to promote the development of the economy and improve the environmental conditions of Honduras. The biomasses explored in this document offer an alternative in areas such as energy production as an alternative to the energies of fossil sources; direct use as material for the production of food and separation processes; extraction or synthesis of chemical substances that can be applied in the chemical industry, medicine, and biotechnology. Currently, the eldest use of these residues in Honduras is in energy production, mainly by direct burning and, to a lesser extent, by a chemical transformation process.
Acknowledgments: The authors thank Diana Medina and Julio Hernández for the technical visit to the Aguilar Paz Research Center of IHCAFE. To Rosario Ortiz and Javier Gutiérrez for the technical visit to the sugar mill Tres Valles S.A. To Javier Amador from ANDAH for the data provided on exports of the different shrimp presentations and to Ángel Marcia for his collaboration in the description of the shrimp production process. To Oscar Andrés Pineda, Alejandro José Cuevas, and Jezoar Etnan Aguilar for translating parts of this document from Spanish to English.
Conflicts of Interest: «The authors declare no conflict of interest.»
REFERENCES
(1) Banco Central de Honduras. Informe de Comercio Exterior de Mercancías Generales; Tegucigalpa, 2021.
(2) Dórea, J. G.; da Costa, T. H. M. Is Coffee a Functional Food? Br. J. Nutr. 2005, 93 (6), 773–782. https://doi.org/10.1079/bjn20051370.
(3) Van Dam, R. M.; Hu, F. B.; Willett, W. C. Coffee, Caffeine, and Health. N. Engl. J. Med. 2020, 383 (4), 369–378. https://doi.org/10.1056/nejmra1816604.
(4) Briandet, R.; Kemsley, E. K.; Wilson, R. H. Discrimination of Arabica and Robusta in Instant Coffee by Fourier Transform Infrared Spectroscopy and Chemometrics. J. Agric. Food Chem. 1996, 44 (1), 170–174. https://doi.org/10.1021/jf950305a.
(5) Keidel, A.; Von Stetten, D.; Rodrigues, C.; Máguas, C.; Hildebrandt, P. Discrimination of Green Arabica and Robusta Coffee Beans by Raman Spectroscopy. J. Agric. Food Chem. 2010, 58 (21), 11187–11192. https://doi.org/10.1021/jf101999c.
(6) Casal, S.; Alves, M. R.; Mendes, E.; Oliveira, M. B. P. P.; Ferreira, M. A. Discrimination between Arabica and Robusta Coffee Species based on Their Amino Acid Enantiomers. J. Agric. Food Chem. 2003, 51 (22), 6495–6501. https://doi.org/10.1021/jf034354w.
(7) Bertrand, B.; Guyot, B.; Anthony, F.; Lasherme, P. Impact of the Coffea Canephora Gene Introgression on Beverage Quality of C. Arabica. Theor. Appl. Genet. 2003, 107 (3), 387–394. https://doi.org/10.1007/s00122-003-1203-6.
(8) Esquivel, P.; Jiménez, V. M. Functional Properties of Coffee and Coffee By-Products. Food Res. Int. 2012, 46 (2), 488–495. https://doi.org/10.1016/j.foodres.2011.05.028.
(9) Organización Internacional de Café. Informe Del Mercado de Café: Enero 2022; 2022.
(10) Organización Internacional de Café. Informe Del Mercado de Café: Agosto 2021; 2021.
(11) IHCAFE. Informe Estadístico 2020-2021; 2021.
(12) IHCAFE. Boletín Estadístico Sobre Café 2015 – 2019; Honduras, 2020.
(13) Davis, A. P.; Govaerts, R.; Bridson, D. M.; Stoffelen, P. An Annotated Taxonomic Conspectus of the Genus Coffea (Rubiaceae). Bot. J. Linn. Soc. 2006, 152 (4), 465–512. https://doi.org/10.1111/j.1095-8339.2006.00584.x.
(14) Ovalle-Rivera, O.; Läderach, P.; Bunn, C.; Obersteiner, M.; Schroth, G. Projected Shifts in Coffea Arabica Suitability among Major Global Producing Regions Due to Climate Change. PLOS One 2015, 10 (4), 1–13. https://doi.org/10.1371/journal.pone.0124155.
(15) Clifford, M. N., and Willson, K. C. Coffee: Botany, Biochemistry and Production of Beans and Beverage; The AVI Publishing Company, Inc.: Westport, Connecticut, 1985.
(16) Soto, C.; Agencia Española de Cooperación Internacional para el Desarrollo; Instituto Interamericano de Cooperación para la Agricultura; Programa Cooperativo Regional para el Desarrollo Tecnológico y Modernización de la Caficultura. Guía Técnica Para El Beneficiado de Café Protegido Bajo Una Indicación Geográfica o Denominación de Origen; IICA, 2010.
(17) IHCAFE. Análisis de La Cadena Del Valor de Café; 2018.
(18) Farah, A. Coffee: Production, Quality and Chemistry. In Coffee; Royal Society of Chemistry, 2019; pp P001–P004. https://doi.org/10.1039/9781782622437-fp001.
(19) Iriondo-DeHond, A.; Iriondo-DeHond, M.; del Castillo, M. D. Applications of Compounds from Coffee Processing By-Products. Biomolecules 2020, 10 (9). https://doi.org/10.3390/biom10091219.
(20) Badwaik, L. S.; Aguilar, C. N.; Haghi, A. K. Food Loss and Waste Reduction; 2021. https://doi.org/10.1201/9781003083900.
(21) Gouvea, B. M.; Torres, C.; Franca, A. S.; Oliveira, L. S.; Oliveira, E. S. Feasibility of Ethanol Production from Coffee Husks. Biotechnol. Lett. 2009, 31 (9), 1315–1319. https://doi.org/10.1007/s10529-009-0023-4.
(22) Murthy, P. S.; Naidu, M. M.; Srinivas, P. Production of α-Amylase under Solid-State Fermentation Utilizing Coffee Waste. J. Chem. Technol. Biotechnol. 2009, 84 (8), 1246–1249. https://doi.org/10.1002/jctb.2142.
(23) Murthy, P. S.; Naidu, M. M. Recovery of Phenolic Antioxidants and Functional Compounds from Coffee Industry By-Products. Food Bioprocess Technol. 2012, 5 (3), 897–903. https://doi.org/10.1007/s11947-010-0363-z.
(24) Hartati, I.; Riwayati, I.; Kurniasari, L. Potential Production of Food Colorant from Coffee Pulp. Ojs2.Unwahas.Ac.Id 2011, No. December 2015, 66–71.
(25) Prata, E. R. B. A.; Oliveira, L. S. Fresh Coffee Husks as Potential Sources of Anthocyanins. LWT – Food Sci. Technol. 2007, 40 (9), 1555–1560. https://doi.org/10.1016/j.lwt.2006.10.003.
(26) Metz, R.; Data, R. U. S. A. ( 12 ) Patent Application Publication ( 10 ) Pub . No .: US 2015 / 0334785 A1 Patent Application Publication. 2015, 1 (19).
(27) Moreno, J.; Cozzano, S.; Mercedes Pérez, A.; Arcia, P.; Curutchet, A. Coffee Pulp Waste as a Functional Ingredient: Effect on Salty Cookies Quality. J. Food Nutr. Res. 2019, 7 (9), 632–638. https://doi.org/10.12691/jfnr-7-9-2.
(28) Rios, M. B.; Iriondo-dehond, A.; Iriondo-dehond, M.; Herrera, T.; Dolores, M. Physicochemical , Nutritional and Sensory Properties. Molecules 2020, No. Cc, 1–16.
(29) Iriondo-DeHond, A.; Elizondo, A. S.; Iriondo-DeHond, M.; Ríos, M. B.; Mufari, R.; Mendiola, J. A.; Ibañez, E.; del Castillo, M. D. Assessment of Healthy and Harmful Maillard Reaction Products in a Novel Coffee Cascara Beverage: Melanoidins and Acrylamide. Foods 2020, 9 (5), 1–18. https://doi.org/10.3390/foods9050620.
(30) Oliveira, W. E.; Franca, A. S.; Oliveira, L. S.; Rocha, S. D. Untreated Coffee Husks as Biosorbents for the Removal of Heavy Metals from Aqueous Solutions. J. Hazard. Mater. 2008, 152 (3), 1073–1081. https://doi.org/10.1016/j.jhazmat.2007.07.085.
(31) Saenger, M.; Hartge, E. U.; Werther, J.; Ogada, T.; Siagi, Z. Combustion of Coffee Husks. Renew. Energy 2001, 23 (1), 103–121. https://doi.org/10.1016/S0960-1481(00)00106-3.
(32) TechnoServe. Manual Técnico de Palma Africana; San Pedro Sula, 2009.
(33) Pamin, K.; Chin, C. W.; Lee, C. H.; Rajanaidu, N.; Corley, R. H. V. Yield Potential in Oil Palm. In Yield potential of planting material and reassessment of oil palm breeding program in Indonesia; Palm Oil Research Institute of Malaysia: Phuket, 1990; pp 11–23.
(34) Zulkifli, H.; Haliman, M.; Chan, K.; Choo, Y.; Mohd Basri, W. Life Cycle Assessment for Oil Palm Fresh Fruit Bunch Production from Continued Land Use for Oil Palm Planted on Mineral Soil (Part 2). J. Oil Palm Res. 2010, 22, 887–894.
(35) Forster, B. P.; Sitepu, B.; Setiawati, U.; Kelanaputra, E. S.; Nur, F.; Rusfiandi, H.; Rahmah, S.; Ciomas, J.; Anwar, Y.; Bahri, S.; Caligari, P. D. S. Oil Palm (Elaeis guineensis). In Genetic Improvement of Tropical Crops; Springer International Publishing, 2017; pp 241–290. https://doi.org/10.1007/978-3-319-59819-2_8.
(36) INDUAGRO. Proceso Productivo de Aceite de Palma. http://www.induagro.com.mx/HOMEAP/ProcProductAP/ProcProductAP.html (accessed 2022-05-24).
(37) Nagendran, B.; Unnithan, U.; Choo, Y.; Sundram, K. Characteristics of Red Palm Oil, a Carotene- andvitamin E–Rich Refined Oil for Food Uses. Food Nutr. Bull. 2000, 21 (2), 189–194.
(38) Delgado, F. Descripción detallada del proceso de extracción de aceite de palma mejorado. https://propalma.webcindario.com/procesomejorado.htm (accessed 2022-05-24).
(39) FAS-USDA. Oilseeds: World Markets and Trade. https://www.fas.usda.gov/data/oilseeds-world-markets-and-trade (accessed 2022-05-27).
(40) UPEG. Palma Africana – Análisis de Coyuntura; 2020.
(41) Osorio, C. E. Uso Integral de La Biomasa de Palma de Aceite. Palmas 2013, 34 (Especial, Tomo II), 315–323.
(42) Nasution, M. A.; Herawan, T.; Rivani, M. Analysis of Palm Biomass as Electricity from Palm Oil Mills in North Sumatera. In Energy Procedia; Elsevier Ltd, 2014; Vol. 47, pp 166–172. https://doi.org/10.1016/j.egypro.2014.01.210.
(43) Subramanian, V.; Chin, C. M.; Ngan, M. A. Energy Database of the Oil Palm. Palm Oil Eng. Bull. 2004, No. 70, 15–21.
(44) Rupilius, W.; Ahmad, S. Palm Oil and Palm Kernel Oil as Raw Materials for Basic Oleochemicals and Biodiesel. Eur. J. Lipid Sci. Technol. 2007, 109 (4), 433–439. https://doi.org/10.1002/ejlt.200600291.
(45) Pineda Ayala, D. M.; Durán Herrera, J. E. Evaluation of Oil Palm Empty Fruit Bunch Wastes as Adsorbent for the Removal of Reactive Dyes from Aqueous Solutions. Ing. Investig. y Tecnol. 2019, 20 (1), 1–9. https://doi.org/10.22201/fi.25940732e.2019.20n1.008.
(46) Maluin, F. N.; Hussein, M. Z.; Idris, A. S. An Overview of the Oil Palm Industry: Challenges and Some Emerging Opportunities for Nanotechnology Development. Agronomy. MDPI AG 2020. https://doi.org/10.3390/agronomy10030356.
(47) Sasson, A.; Malpica, C. Bioeconomy in Latin America. N. Biotechnol. 2018, 40, 40–45. https://doi.org/10.1016/J.NBT.2017.07.007.
(48) Peter, R. Ingeniería de La Caña de Azúcar, 2nd ed.; Verla Dr. Albert Bartens KG: Berlin, 2012.
(49) Lizandro, L.; Montejo, D. Manual de Producción de Caña de Azúcar (Saccharum officinarum L.) Manual de Producción de Caña de Azúcar (Saccharum officinarum L.). 2002, 148.
(50) Enma M. Manals-Cutiño, Margarita Penedo-Medina, D. S.-T. Caracterización Del Bagazo de Caña Como Biomasa Vegetal. Tecnol. Química 2015, 35 (2224–6185), 179–192.
(51) Lagos-Burbano, E.; Castro-Rincón, E. Sugar Cane and By-Products of the Sugar Agro-Industry in Ruminant Feeding: A Review. Agron. Mesoamerican 2019, 30 (3), 917–934. https://doi.org/10.15517/am.v30i3.34668.
(52) García-Torres, R.; Rios-Leal, E.; Martínez-Toledo, Á.; Ramos-Morales, F. R.; Cruz-Sanchez, J. S.; del María, C. C. D. Uso de Cachaza y Bagazo de Caña de Azúcar En La Remoción de Hidrocarburos En Suelo Contaminado. Rev. Int. Contam. Ambient. 2011, 27 (1), 31–39.
(53) Ada, I.; Cerna, S. Energías Renovables En Honduras; 2019.
(54) Secretaría de Estado en el Despacho de Energía. Balance Energético Nacional. 2017, 1.
(55) Secretaría de Estado en el Despacho de Energía. Energético. 2018, 1.
(56) Secretaría de Estado en el Despacho de Energía. Balance Energético Nacional 2019. 2019, 99.
(57) Secretaría de Estado en el Despacho de Energía. Balance Energético Nacional. 2020, 1.
(58) Barroso, J.; Barreras, F.; Amaveda, H.; Lozano, A. On the Optimization of Boiler Efficiency Using Bagasse as Fuel. Fuel 2003, 82 (12), 1451–1463. https://doi.org/10.1016/S0016-2361(03)00061-9.
(59) Mbohwa, C. Modelling Bagasse Electricity Generation: An Application to the Sugar Industry in Zimbabwe. Proc. from Int. Conf. Adv. Eng. Technol. 2006, 354–367. https://doi.org/10.1016/B978-008045312-5/50040-6.
(60) Deepchand, K. Deepchand: Bagasse energy cogeneration in Mauritius… – Google Académico. https://scholar.google.com/scholar?cluster=16318600630032801286&hl=es&as_sdt=2005&sciodt=0,5 (accessed 2022-05-05).
(61) Murefu, M. Steam Reticulation at Hippo Valley Sugar Estates,… – Google Académico. https://scholar.google.com/scholar?hl=es&as_sdt=0%2C5&q=Steam+Reticulation+at+Hippo+Valley+Sugar+Estates%2C+Publication+of+the+Department+of+Mechanical+Engineering&btnG= (accessed 2022-05-05).
(62) A, M. Case Study on Steam Raising: A complete energy balance… – Google Académico. https://scholar.google.com/scholar?hl=es&as_sdt=0%2C5&q=Case+Study+on+Steam+Raising%3A+A+complete+energy+balance+for+Triangle+Sugar+Limited&btnG= (accessed 2022-05-05).
(63) Shorewala, V. Thermal Efficiency of Bagasse As Compared to Coal. SSRN Electron. J. 2020. https://doi.org/10.2139/ssrn.3591125.
(64) Garcia-Perez, M.; Chaala, A.; Pakdel, H.; Roy, C. Sugarcane Bagasse Vacuum Pyrolysis. J. Anal. Appl. Pyrol. 2002, 65 (March 2019), 111–136.
(65) Hussein, A. A. E.; Shafiq, N.; Nuruddin, M. F.; Memon, F. A. Compressive Strength and Microstructure of Sugar Cane Bagasse Ash Concrete. Res. J. Appl. Sci. Eng. Technol. 2014, 7 (12), 2569–2577. https://doi.org/10.19026/rjaset.7.569.
(66) Mangi, S. A.; Jamaluddin, N.; Wan Ibrahim, M. H.; Abdullah, A. H.; Abdul Awal, A. S. M.; Sohu, S.; Ali, N. Utilization of Sugarcane Bagasse Ash in Concrete as Partial Replacement of Cement. In IOP Conference Series: Materials Science and Engineering; Institute of Physics Publishing, 2017; Vol. 271. https://doi.org/10.1088/1757-899X/271/1/012001.
(67) International, A. M.-C.; 1994, undefined. High-Reactivity Metakaolin; a New Generation Mineral Admixture. ci.nii.ac.jp.
(68) Bonilla, M.; Borrachero, M. V.; Monzó, J. M.; Akasaki, J. L.; Ma-Tay, D.; Payá, J. Study of Pozzolanic Properties of Two Sugarcane Bagasse Ash Samples from Honduras. In Key Engineering Materials; Trans Tech Publications Ltd, 2016; Vol. 668, pp 357–366. https://doi.org/10.4028/www.scientific.net/KEM.668.357.
(69) Chandel, A. K.; Albarelli, J. Q.; Santos, D. T.; Chundawat, S. P.; Puri, M.; Meireles, M. A. A. Comparative Analysis of Key Technologies for Cellulosic Ethanol Production from Brazilian Sugarcane Bagasse at a Commercial Scale. Biofuels, Bioprod. Biorefining 2019, 13 (4), 994–1014. https://doi.org/10.1002/bbb.1990.
(70) Harris, P. V.; Xu, F.; Kreel, N. E.; Kang, C.; Fukuyama, S. New Enzyme Insights Drive Advances in Commercial Ethanol Production. Curr. Opin. Chem. Biol. 2014, 19 (1), 162–170. https://doi.org/10.1016/J.CBPA.2014.02.015.
(71) Losordo, Z.; Mcbride, J.; Rooyen, J. Van; Wenger, K.; Willies, D.; Froehlich, A. Modeling and Analysis Cost Competitive Second-Generation Ethanol Production from Hemicellulose in a Brazilian Sugarcane Biorefi Nery. Biofuels, Bioprod. Bioref 2016, 10, 589–602. https://doi.org/10.1002/bbb.1663.
(72) Schmitt, C. C.; Moreira, R.; Neves, R. C.; Richter, D.; Funke, A.; Raffelt, K.; Grunwaldt, J. D.; Dahmen, N. From Agriculture Residue to Upgraded Product: The Thermochemical Conversion of Sugarcane Bagasse for Fuel and Chemical Products. Fuel Process. Technol. 2020, 197, 106199. https://doi.org/10.1016/J.FUPROC.2019.106199.
(73) Cruz, Rolando; Dopico, Daiysi; Figueredo, José; Rodríguez, Maira; Martínez, G. Lignina de Bagazo Con Fines Medicinales.Pdf. Rev. Med. Exp. INS 1997, XIV, 5.
(74) Sakagami, H.; Kushida, T.; Oizumi, T.; Nakashima, H.; Makino, T. Distribution of Lignin–Carbohydrate Complex in Plant Kingdom and Its Functionality as Alternative Medicine. Pharmacol. Ther. 2010, 128 (1), 91–105. https://doi.org/10.1016/J.PHARMTHERA.2010.05.004.
(75) Hernández, Evelyn; Samayoa, Svetlana; Álvarez, Erwin; Talavera, C. Iniciativas De Biogás. SNV 2012, 1 (1).
(76) González García, Y.; Reynoso, O. G.; Arellano, J. N. Potencial Del Bagazo de Agave Tequilero Para La Producción de Biopolímeros y Carbohidrasas Por Bacterias Celulolíticas y Para La Obtención de Compuestos Fenólicos. 2005.
(77) Nirmal, N. P.; Santivarangkna, C.; Rajput, M. S.; Benjakul, S. Trends in Shrimp Processing Waste Utilization: An Industrial Prospective. Trends Food Sci. Technol. 2020, 103 (May), 20–35. https://doi.org/10.1016/j.tifs.2020.07.001.
(78) FAO. The State of World Fisheries and Aquaculture 2022; FAO: Rome, Italy, 2022. https://doi.org/10.4060/cc0461en.
(79) SICA. Sistema Integrado de Información Estadística SICA si-ESTAD. www.sica.int/si-estad/clasificacion/estadistica/?indicatorIds=I362d1.
(80) Camarón de Honduras – ANDAH. https://andah.hn/camaron-de-honduras/ (accessed 2022-02-17).
(81) Exportaciones de Camarón Honduras – ANDAH. https://andah.hn/exportaciones-de-camaron-honduras/ (accessed 2022-02-17).
(82) Gracia, A.; Díaz, J. M. Libro Rojo de Los Invertebrados Marinos de Colombia: Litopenaeus vannamei; Bogota, 2002.
(83) Valles-Jimenez, R.; Cruz, P.; Perez-Enriquez, R. Population Genetic Structure of Pacific White Shrimp (Litopenaeus Vannamei) from Mexico to Panama: Microsatellite DNA Variation. Mar. Biotechnol. (NY). 2004, 6, 475–484. https://doi.org/10.1007/s10126-004-3138-6.
(84) Haws, M. C.; Boyd, C. E.; Green, B. W. Buenas Prácticas de Manejo En El Cultivo de Camarón En Honduras; 2001.
(85) Dugassa, H.; Gaetan, D. Biology of White Leg Shrimp, Penaeus vannamei: Review; 2018.
(86) Integración del Sector Acuícola de Honduras – ANDAH. https://andah.hn/integracion-del-sector-acuicola-de-honduras/ (accessed 2022-02-27).
(87) ANDAH, A. J. Exportación de Camarón En Honduras En Diferentes Persentaciones; Choluteca, 2022.
(88) ANDAH, A. J. Producción de Camarón En Honduras; Choluteca, 2022.
(89) Duan, S.; Zhang, Y. X.; Lu, T. T.; Cao, D. X.; Chen, J. D. Shrimp Waste Fermentation Using Symbiotic Lactic Acid Bacteria. In Advanced Engineering Materials; Advanced Materials Research; Trans Tech Publications Ltd, 2011; Vol. 194, pp 2156–2163. https://doi.org/10.4028/www.scientific.net/AMR.194-196.2156.
(90) Yan, N.; Chen, X. Don’t Waste Seafood Waste: Turning Cast-off Shells into Nitrogen-Rich Chemicals Would Benefit Economies and the Environment. Nature 2015, 524, 155–157.
(91) Fernandez, J. G.; Ingber, D. E. Manufacturing of Large-Scale Functional Objects Using Biodegradable Chitosan Bioplastic. Macromol. Mater. Eng. 2014, 299 (8), 932–938. https://doi.org/https://doi.org/10.1002/mame.201300426.
(92) Suryawanshi, N.; Eswari, J. S. Shrimp Shell Waste as a Potential Raw Material for Biorefinery—a Revisit. Biomass Convers. Biorefinery 2022, 12 (5), 1977–1984. https://doi.org/10.1007/s13399-020-01271-2.
(93) Shamshina, J. L.; Berton, P.; Rogers, R. D. Advances in Functional Chitin Materials: A Review. ACS Sustain. Chem. Eng. 2019, 7 (7), 6444–6457. https://doi.org/10.1021/acssuschemeng.8b06372.
(94) Jayakumar, R.; Menon, D.; Manzoor, K.; Nair, S. V; Tamura, H. Biomedical Applications of Chitin and Chitosan Based Nanomaterials—A Short Review. Carbohydr. Polym. 2010, 82 (2), 227–232. https://doi.org/https://doi.org/10.1016/j.carbpol.2010.04.074.
(95) Gómez-Estaca, J.; Alemán, A.; López-Caballero, M. E.; Baccan, G. C.; Montero, P.; Gómez-Guillén, M. C. Bioaccessibility and Antimicrobial Properties of a Shrimp Demineralization Extract Blended with Chitosan as Wrapping Material in Ready-to-Eat Raw Salmon. Food Chem. 2019, 276, 342–349. https://doi.org/https://doi.org/10.1016/j.foodchem.2018.10.031.
(96) Mathew, G. M.; Mathew, D. C.; Sukumaran, R. K.; Sindhu, R.; Huang, C.-C.; Binod, P.; Sirohi, R.; Kim, S.-H.; Pandey, A. Sustainable and Eco-Friendly Strategies for Shrimp Shell Valorization. Environ. Pollut. 2020, 267, 115656. https://doi.org/https://doi.org/10.1016/j.envpol.2020.115656.
(97) Gómez-Guillén, M. C.; Montero, P.; López-Caballero, M. E.; Baccan, G. C.; Gómez-Estaca, J. Bioactive and Technological Functionality of a Lipid Extract from Shrimp (L. vannamei) Cephalothorax. LWT – Food Sci. Technol. 2018, 89, 704–711. https://doi.org/10.1016/j.lwt.2017.11.052.
(98) Zagalsky, P. F. [9] Invertebrate Carotenoproteins; 1985; Vol. 111. https://doi.org/10.1016/S0076-6879(85)11011-6.
(99) Kandra, P.; Challa, M. M.; Kalangi Padma Jyothi, H. Efficient Use of Shrimp Waste: Present and Future Trends. Appl. Microbiol. Biotechnol. 2012, 93 (1), 17–29. https://doi.org/10.1007/s00253-011-3651-2.
(100) De Holanda, H. D.; Netto, F. M. Recovery of Components from Shrimp (Xiphopenaeus Kroyeri) Processing Waste by Enzymatic Hydrolysis. J. Food Sci. 2006, 71 (5), 298–303. https://doi.org/10.1111/j.1750-3841.2006.00040.x.
(101) Guerin, M.; Huntley, M. E.; Olaizola, M. Haematococcus Astaxanthin: Applications for Human Health and Nutrition. Trends Biotechnol. 2003, 21 (5), 210–216. https://doi.org/10.1016/S0167-7799(03)00078-7.
(102) Kannan, S.; Gariepy, Y.; Raghavan, G. S. V. Optimization and Characterization of Hydrochar Derived from Shrimp Waste. Energy and Fuels 2017, 31 (4), 4068–4077. https://doi.org/10.1021/acs.energyfuels.7b00093.
(103) Galdámez Cáceres, A. Guía Técnica Del Cultivo Del Marañón. 2004, 1 (503), 1–69.
(104) Rico, R.; Bulló, M.; Salas-salvadó, J. Nutritional Composition of Raw Fresh Cashew (Anacardium occidentale L.) Kernels from Different Origin. Food Sci. Nutr. 2015, 329–338. https://doi.org/10.1002/fsn3.294.
(105) Ministerio de Agricultura y Ganadería, M. Boletín Mercado de La Nuez de Marañón. 2002, 1–20.
(106) Oliveira, N. N.; Mothé, C. G.; Mothé, M. G.; de Oliveira, L. G. Cashew Nut and Cashew Apple: A Scientific and Technological Monitoring Worldwide Review. J. Food Sci. Technol. 2020, 57 (1), 12–21. https://doi.org/10.1007/s13197-019-04051-7.
(107) Afanasjeva, N.; Castillo, L. C.; Sinisterra, J. C. Lignocellulosic Biomass. Part I: Biomass Transformation. J. Sci. with Technol. Appl. 2017, 3, 27–43. https://doi.org/10.34294/j.jsta.17.3.22.
(108) Balachandran, V. S.; Jadhav, S. R.; Vemula, P. K.; John, G. Recent Advances in Cardanol Chemistry in a Nutshell: From a Nut to Nanomaterials. Chem. Soc. Rev. 2013, 42 (2), 427–438. https://doi.org/10.1039/c2cs35344j.
(109) Sharma, P.; Gaur, V. K.; Sirohi, R.; Larroche, C.; Kim, S. H.; Pandey, A. Valorization of Cashew Nut Processing Residues for Industrial Applications. Ind. Crops Prod. 2020, 152 (January), 112550. https://doi.org/10.1016/j.indcrop.2020.112550.
(110) Aplícano, V. H.; Rodríguez, M.; Gómez, D.; Domínguez, V. B. y J.-M. D. Análisis Rápido de La Cadena de Valor de Marañon En El Golfo de Fonseca, Honduras. Swisscontact 2014, 1–34.
(111) Secretaría de Agricultura y Ganadería de Honduras. Boletín de Perfil de Mercado Del Marañon y Sus Derivados. 2014, 1–25.
(112) Casaca, Á. D.; Sierra, E.; Cruz, J.; Donaire, R. A.; Talavera, M. J.; Flores, S. Boletín Informativo: El Cultivo Del Marañón (Anacardium occidentale). 2005, 1–12.
(113) Piedrahita, L. R.; Wiesner, L. V. A.; Chavarro, N. A. P. Propuesta de Aprovechamiento Integral de Marañon: Nuez, Pseudofruto y Subproductos. 2011.
(114) Hu, F. B.; Manson, J. A. E.; Willett, W. C. Types of Dietary Fat and Risk of Coronary Heart Disease: A Critical Review. J. Am. Coll. Nutr. 2001, 20 (1), 5–19. https://doi.org/10.1080/07315724.2001.10719008.
(115) Jakappa Pail, P. Indian Cashew Food. Integr. Food, Nutr. Metab. 2017, 4 (2), 1–5. https://doi.org/10.15761/ifnm.1000173.
(116) Fatty Acid and Protein in Cashew Ut.Pdf.
(117) Akinhanmi, T. F.; Atasie, V. N. Volume 2, Issue 1, 2008 Chemical Composition and Physicochemical Properties Of Cashew Nut. J. Agric. Food Environ. Sci. 2008, 2 (1), 1–10.
(118) Oliveira, N. N.; Mothé, C. G.; Mothé, M. G.; de Oliveira, L. G. Cashew Nut and Cashew Apple: A Scientific and Technological Monitoring Worldwide Review. J. Food Sci. Technol. 2020, 57 (1), 12–21. https://doi.org/10.1007/s13197-019-04051-7.
(119) Aremu, M. O.; Olonisakin, A.; Bako, D. A.; Madu, P. C. Compositional Studies and Physicochemical Characteristics of Cashew Nut (Anarcadium Occidentale) Flour. Pakistan J. Nutr. 2006, 5 (4), 328–333. https://doi.org/10.3923/pjn.2006.328.333.
(120) Griffin, L. E.; Dean, L. L. Nutrient Composition of Raw, Dry-Roasted, and Skin-On Cashew Nuts. J. Food Res. 2017, 6 (6), 13. https://doi.org/10.5539/jfr.v6n6p13.
(121) Chávez, F.; Alas, J. R.; Rovira, M. D.; Ramos, E. H. Potencial de La Biomasa Como Fuente de Energía En La Zona de La Bahía de Jiquilisco; 2013.
(122) Caillol, S. Cardanol: A Promising Building Block for Biobased Polymers and Additives. Curr. Opin. Green Sustain. Chem. 2018, 14, 26–32. https://doi.org/10.1016/j.cogsc.2018.05.002.
(123) Sánchez, L.; Chávez, J.; Ríos, L. A.; Cardona, S. M. Evaluación de Un Antioxidante Natural Extraído Del Marañón (Anacardium occidentale L.) Para Mejorar La Estabilidad Oxidativa Del Biodiesel de Jatropha. Inf. Tecnológica 2015, 26 (6), 19–30. https://doi.org/10.4067/S0718-07642015000600004.
(124) Koteich-Khatib, S.; Vivas, J.; Bahsas, A.; Rosales-Oballos, Y.; Bullón, J. Caracterización Por RMN y Actividad Antibacteriana de Los Componentes Del Aceite de La Semilla de Merey (Anacardium Occidentale L.). Av. en Quim. 2019, 14 (1), 31–40.
(125) de Araujo, J. T. C.; Martin-Pastor, M.; Pérez, L.; Pinazo, A.; de Sousa, F. F. O. Development of Anacardic Acid-Loaded Zein Nanoparticles: Physical Chemical Characterization, Stability and Antimicrobial Improvement. J. Mol. Liq. 2021, 332. https://doi.org/10.1016/j.molliq.2021.115808.
(126) Goulart da Silva, G.; de Oliveira Braga, L. E.; Souza de Oliveira, E. C.; Valério Tinti, S.; de Carvalho, J. E.; Goldoni Lazarini, J.; Rosalen, P. L.; Dionísio, A. P.; Tasca Gois Ruiz, A. L. Cashew Apple Byproduct: Gastroprotective Effects of Standardized Extract. J. Ethnopharmacol. 2021, 269 (December 2020). https://doi.org/10.1016/j.jep.2020.113744.
(127) Schultz, D. J.; Wickramasinghe, N. S.; Klinge, C. M. Chapter Six Anacardic Acid Biosynthesis and Bioactivity. Recent Adv. Phytochem. 2006, 40, 1–26.
(128) Báez, R. H.; Jiménez, C. V.; Ubau, M. H.; Baudrit, J. V.; Química, E. De; Nacional, U.; Dengo, C. O.; Rica, C.; Nanotecnología, L. N. De; Conare, L. C.; José, S. Cardanol Revista Iberoamericana de Polímeros. 2019, 20 (6), 262–278.
(129) Lafont, J. J.; Paéz, M. S.; Portacio, A. A. Extracción y Caracterización Fisicoquímica Del Aceite de La Semilla (Almendra) Del Marañón (Anacardium Occidentale L). Inf. Tecnológica 2011, 22 (1), 51–58. https://doi.org/10.4067/S0718-0764201100.
(130) Muthu Dineshkumar, R.; Meera Sheriffa Begum, K. M.; Ramanathan, A. Comprehensive Characterization of Cashew Nutshell for Biomass Gasification. Mater. Today Proc. 2019, 46 (xxxx), 9837–9843. https://doi.org/10.1016/j.matpr.2020.10.932.
Received: 20 June 2023/ Accepted: 25 August 2023 / Published:15 September 2023
Citation: Bulnes D, Melgar S, Vega E, Rubio A, Espinal A, Velásquez-Tinoco D G, Díaz Reyes G, Estrada-Lopez E D, Ortiz-Hernández O V. A look into Honduran biomass: facts, uses and potential applications. Revis Bionatura 2023;8 (3) 37. http://dx.doi.org/10.21931/RB/2023.08.03.37